What is LiDAR and How Does It Work?
In the early 1960s, as lasers debuted, LiDAR emerged - a fusion of "light" and "radar." Initially mapping small water bodies, LiDAR soared in the 1980s with GPS integration, revolutionizing geospatial data collection.
Back then, LiDAR sensors were hefty, tethered to piloted planes, and operated manually, limiting their accessibility. Fast forward to today: LiDAR is compact, cost-effective, and ingrained in diverse sectors, even in iPhones, crafting 3D models from 4.5 meters away.
This 60-year evolution is remarkable. But what is LiDAR, and how does it work? In this guide, we dissect LiDAR's components, explore its applications, and unravel the synergy of laser precision and data analytics
What is LiDAR?
LiDAR, an acronym for "Light Detection and Ranging," stands as a revolutionary distance-measuring sensor, akin to its counterparts radar and sonar. Functioning by emitting laser pulses that rebound off objects, LiDAR sensors meticulously capture the intricate structure of their surroundings. The process involves recording the energy of the reflected light, enabling the precise determination of distances to objects. This, in turn, facilitates the creation of detailed 2D or 3D representations of the environment.
The JOUAV CW-25E VTOL drone with JoLiDAR-LR22S LiDAR sensor
LiDAR's superiority in sensing accuracy, compared to traditional technologies like radar and sonar, stems from its laser precision. It crafts detailed point clouds, essentially translating the features of objects or environments into tangible, solid representations on displays.
Operating across various wavelengths, from infrared (10 micrometers) to ultraviolet (250 nanometers), LiDAR employs different types of scattering (Rayleigh, Raman, Mie) tailored for specific applications. The ability to identify wavelength-dependent changes in the scattering signal extends its utility to the remote mapping of atmospheric composition and object properties.
The versatility of LiDAR extends across multiple platforms, from being mounted on aircraft such as airplanes, helicopters, or drones, to satellites, automobiles, and ground-based or hand-held devices. Remarkably, LiDAR has become so accessible that it's now found in everyday devices, including cell phones.
Types of LiDAR Sensors
There are different types of LiDAR sensors, and they can be broadly categorized based on their technology, application, and deployment. Here are some common types of LiDAR sensors:
Based on Platform
LiDAR sensors can be categorized based on the platform on which they are deployed. This includes aerial and ground-based LiDAR systems.
Airborne LiDAR
Airborne LiDAR systems are mounted on either fixed-wing drones or helicopters, and they play a crucial role in topographic and bathymetric applications.
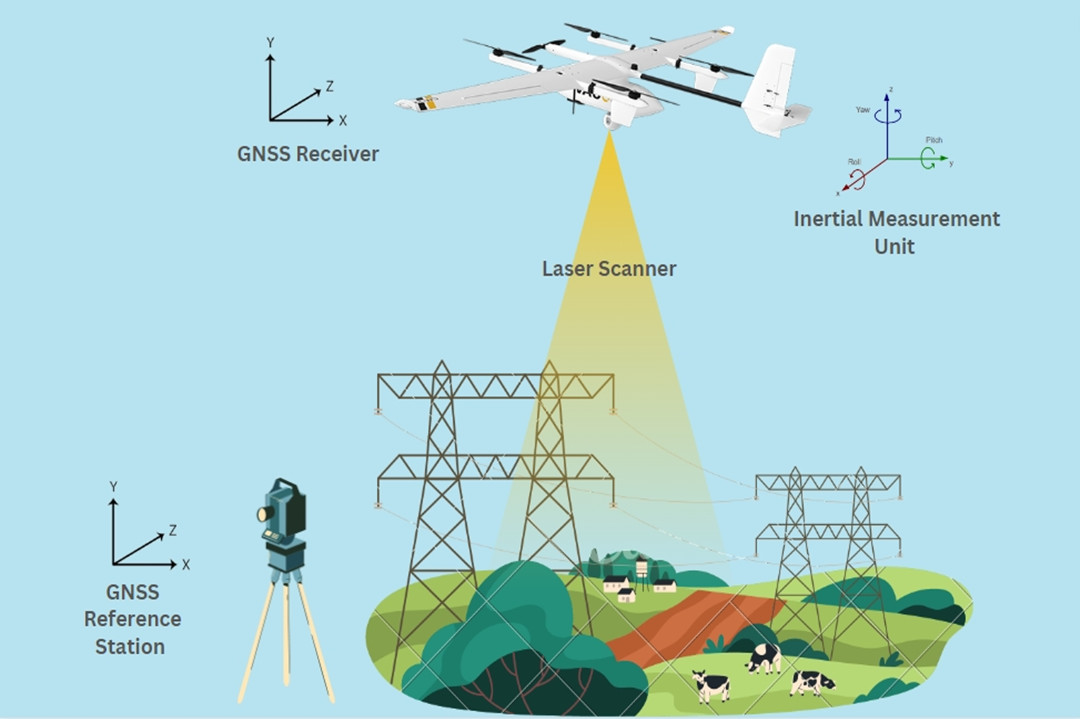
Topographic LiDAR
Topographic LiDAR, employing a 1,064 nm near-infrared laser, is extensively used for land-based mapping. This system captures a dense point cloud, enabling the creation of high-precision 3D maps and models of the Earth's surface. To enhance accuracy, UAV topographic LiDAR often integrates a GPS receiver and an inertial navigation system (INS). Post-processing of data further refines precision.
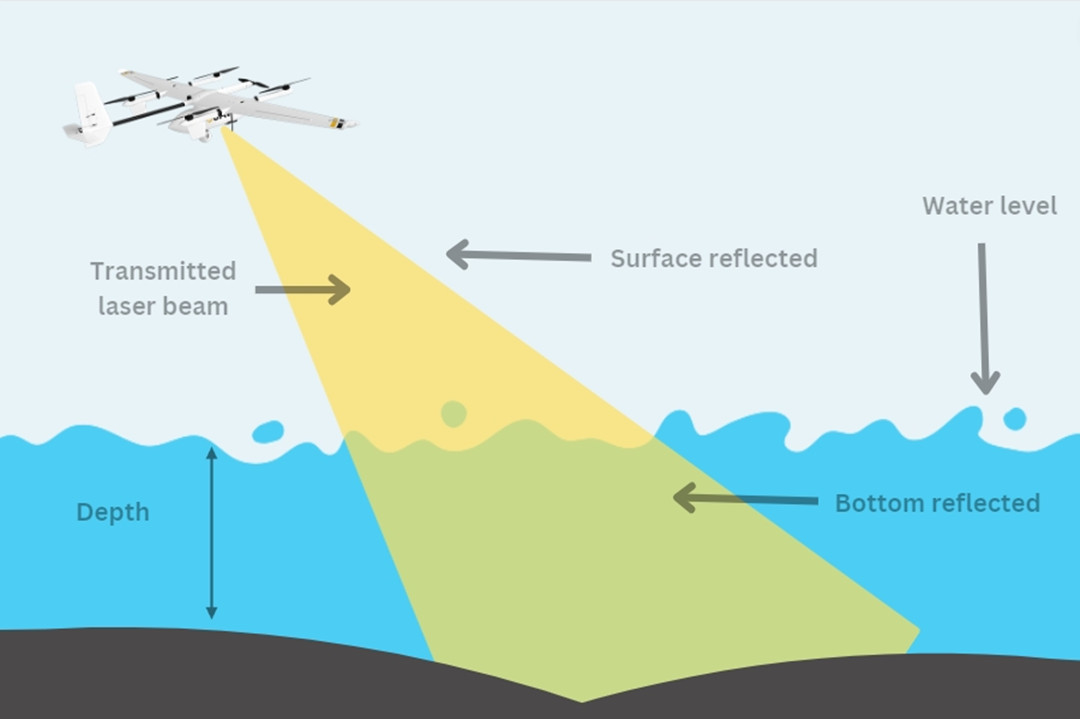
Bathymetric LiDAR
Bathymetric LiDAR systems, utilizing a green wavelength of 532nm, are specifically designed to measure seafloor depths and shoreline elevations. These systems provide valuable information near coastlines, harbors, and shores. The dual functionality involves collecting elevation and water depth simultaneously, utilizing both infrared and green lasers for comprehensive airborne surveys.
Terrestrial LiDAR
Terrestrial LiDAR is mounted on a stationary or mobile platform, such as tripods, vehicles, or handheld devices.
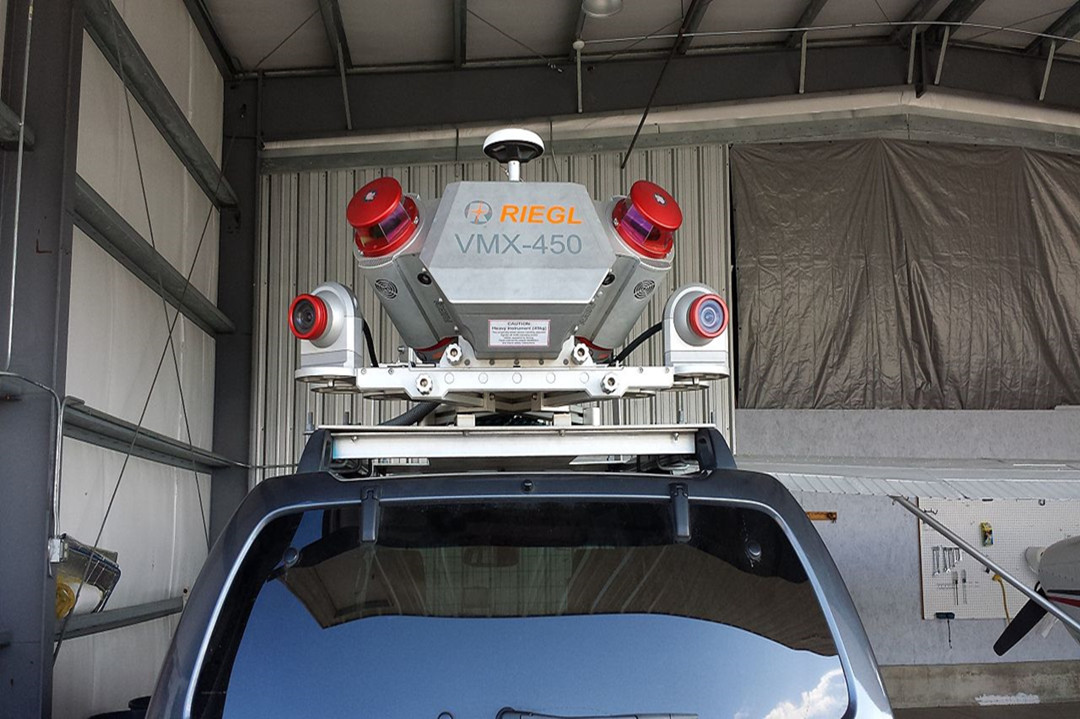
Mobile LiDAR
Dynamically mounted on moving platforms such as vehicles, trains, or boats, Mobile LiDAR systems play a crucial role in analyzing road infrastructure, identifying obstacles, and surveying transportation corridors. These systems often integrate LiDAR sensors with cameras, GPS, and inertial navigation systems for comprehensive data collection.
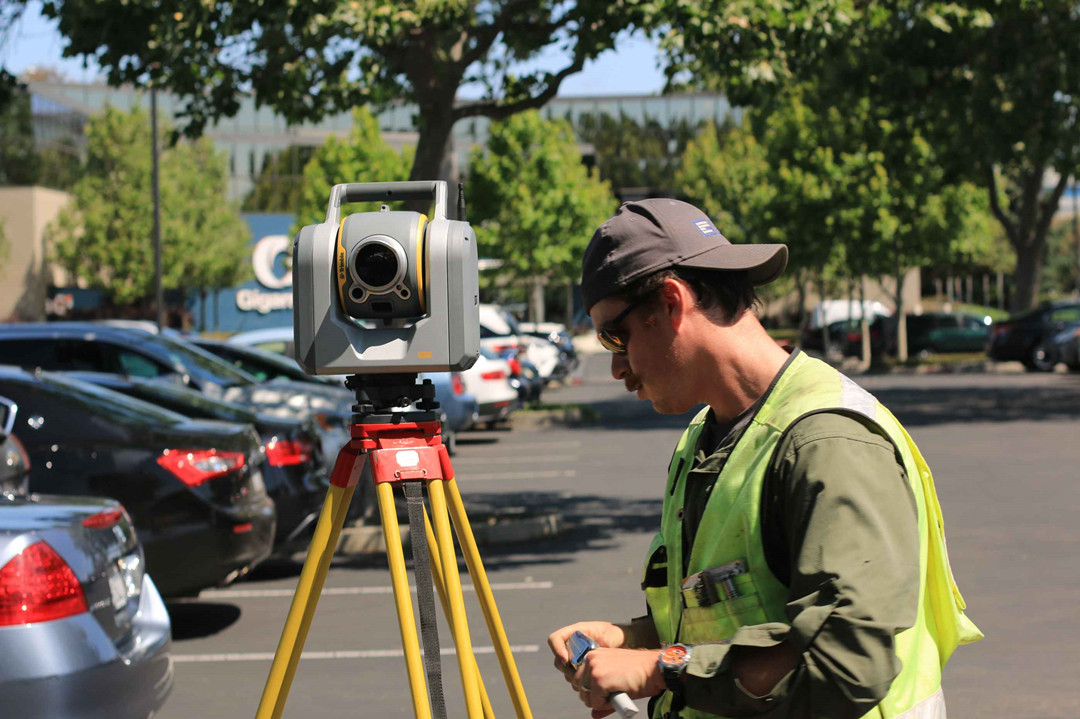
Static LiDAR
Positioned on tripods, Static LiDAR systems bring portability and flexibility to detailed scanning, accommodating both indoor and outdoor environments. Their applications span various industries, including engineering projects, mining operations, land surveying, and archaeological excavations.
Based on Detection Techniques
LiDAR can be categorized into Photon LiDAR and Linear LiDAR based on how they detect and measure reflected light. Linear LiDAR, including single-line variants, is commonly used across industries. Photon LiDAR, especially single-photon variants, offers high sensitivity and accuracy but is less common and more expensive.
Photon LiDAR
Photon LiDAR systems, whether single-photon or multi-photon, operate based on the principle of measuring the time it takes for emitted photons to return after interacting with objects in the environment.
Single-Photon LiDAR works by emitting a single photon at a time and then detecting the time it takes for that photon to return after bouncing off surfaces. This method allows for extremely sensitive detection, especially useful for capturing faint signals from distant or low-reflectivity objects or surfaces. The system calculates distances based on the time delay of the returned photons, enabling precise ranging and mapping.
Multi-Photon LiDAR functions similarly to single-photon LiDAR but requires the detection of multiple photons before registering a return signal. By averaging signals over multiple photon detections, this approach improves signal-to-noise ratio and enhances accuracy. Multi-photon LiDAR is particularly beneficial in environments with high noise levels or complex structures, where single-photon detection might be less effective.
Linear LiDAR
On the other hand, Linear LiDAR systems, whether single-line or multiple-line, operate based on the scanning pattern of their laser beams.
Single-Line LiDAR emits a single laser beam in a straight line, which is then rotated or oscillated to cover a larger area. As the beam hits objects in its path, it measures the time it takes for the reflected light to return. This method provides basic distance measurements along a single line, suitable for simple mapping and obstacle detection tasks.
Multiple-Line LiDAR emits multiple laser beams simultaneously, often arranged in a vertical or horizontal array. These beams scan the environment concurrently, capturing more data points in a single scan compared to single-line LiDAR. This approach increases data density and coverage, enabling faster data acquisition and more detailed 3D mapping. Multiple-line LiDAR is advantageous for applications requiring high-resolution mapping, such as autonomous vehicle navigation and urban planning.
Based on Scanning Methods
LiDAR sensors employ different scanning methods to capture information about the surroundings. The scanning method employed greatly influences the data resolution and coverage area.
Solid-State LiDAR
Solid-state LiDAR, which includes technologies such as Micro-Electro-Mechanical Systems (MEMS) and Optical Phase Arrays (OPA), operates without any moving parts. MEMS-based LiDAR employs tiny mirrors or other microstructures to direct laser beams, while OPA relies on electronically controlled phase shifting of light. While MEMS and OPA offer solid-state solutions, they may not cover a full 360°, so multiple LiDARs are often used in self-driving cars for comprehensive coverage. OPA is less deployed and hasn't been extensively tested in self-driving cars at scale.
Mechanical LiDAR
Mechanical LiDAR, in contrast, employs physical movement, often relying on spinning mirrors or other mechanical components to direct laser beams. The rotational movement enables a broader field of view, facilitating comprehensive environmental scanning. Advantages of Mechanical LiDAR include longer range capabilities and higher resolution, making them suitable for applications requiring detailed and long-distance object detection. However, their mechanical components introduce greater complexity and potential points of failure.
Flash LiDAR
Flash LiDAR, also known as Flash LiDAR Imaging or Time-of-Flight (ToF) LiDAR, operates by emitting a broad beam of light and capturing the reflected signals in a single flash. This allows for the simultaneous measurement of the entire scene, providing a rapid and comprehensive 3D representation. Flash LiDAR excels in capturing dynamic scenes quickly and comprehensively, making it suitable for applications like autonomous vehicles, robotics, and augmented reality. However, it may have limitations in terms of range compared to some mechanical LiDAR systems.
Based on Dimensionality
LiDAR sensors are categorized based on the dimensionality of the data they capture. This classification helps determine the level of detail and precision achievable in a given application.
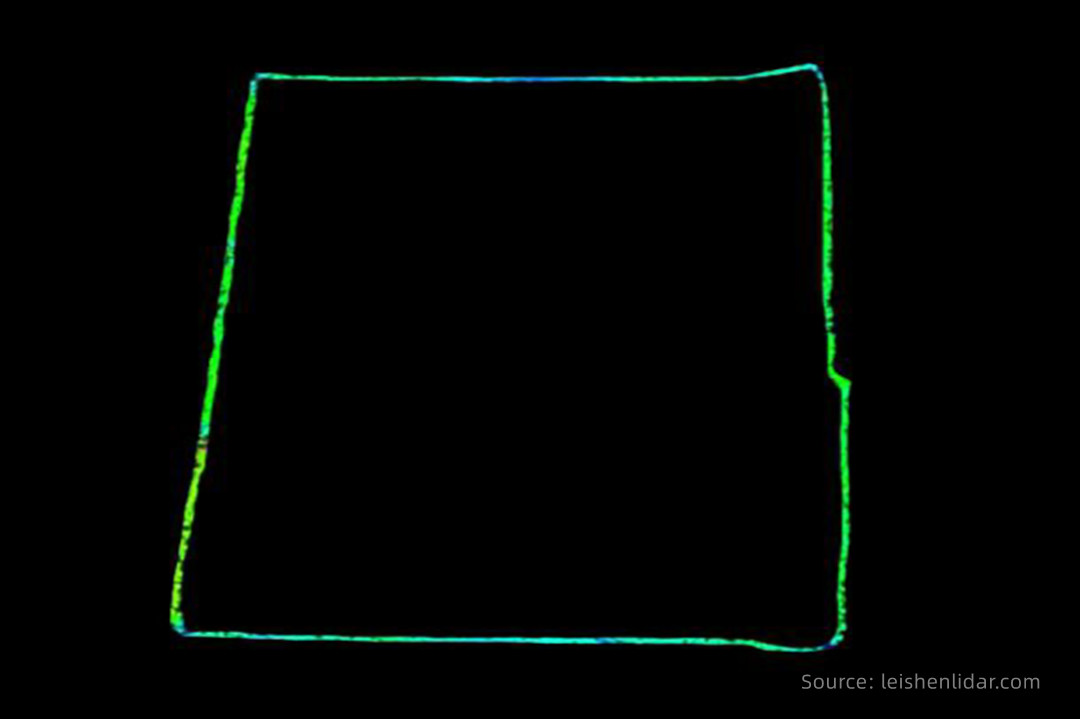
2D LiDAR
2D LiDAR operates by emitting laser pulses in a single plane, typically horizontal or vertical. The sensor gauges the time it takes for the laser pulses to return, offering distance information within that specific plane. This design comprises an emitter laser diode and a receiver photodiode, with each color representing a distinct "layer." For instance, a 64-layer LiDAR sends 64 lines of photons. This type of LiDAR finds utility in applications where a flat representation of the environment suffices, such as in industrial automation for basic obstacle detection, inventory management, and fundamental mapping tasks.
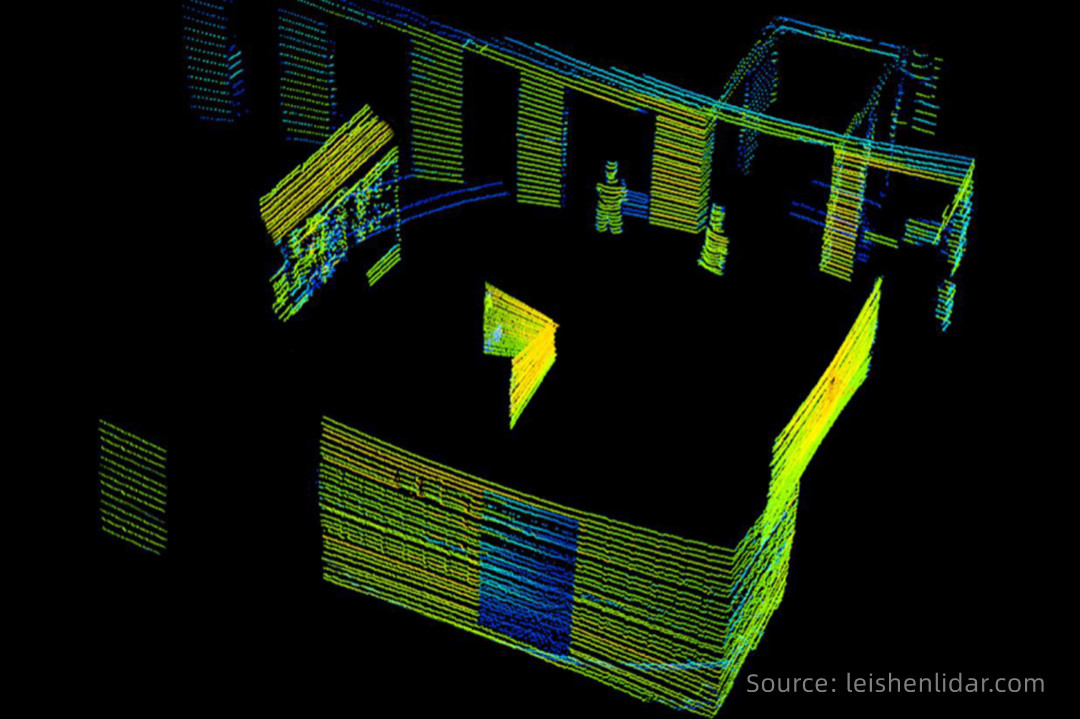
3D LiDAR
3D LiDAR captures spatial information in multiple planes (X, Y, Z), generating a detailed three-dimensional point cloud. This is achieved either by employing multiple lasers or a scanning mechanism that steers the laser beam in both horizontal and vertical directions. Ideal for applications demanding a thorough understanding of the three-dimensional structure of the environment, 3D LiDAR is commonly used in autonomous vehicles for obstacle avoidance, mapping, navigation, as well as in urban planning, forestry, and archaeology.
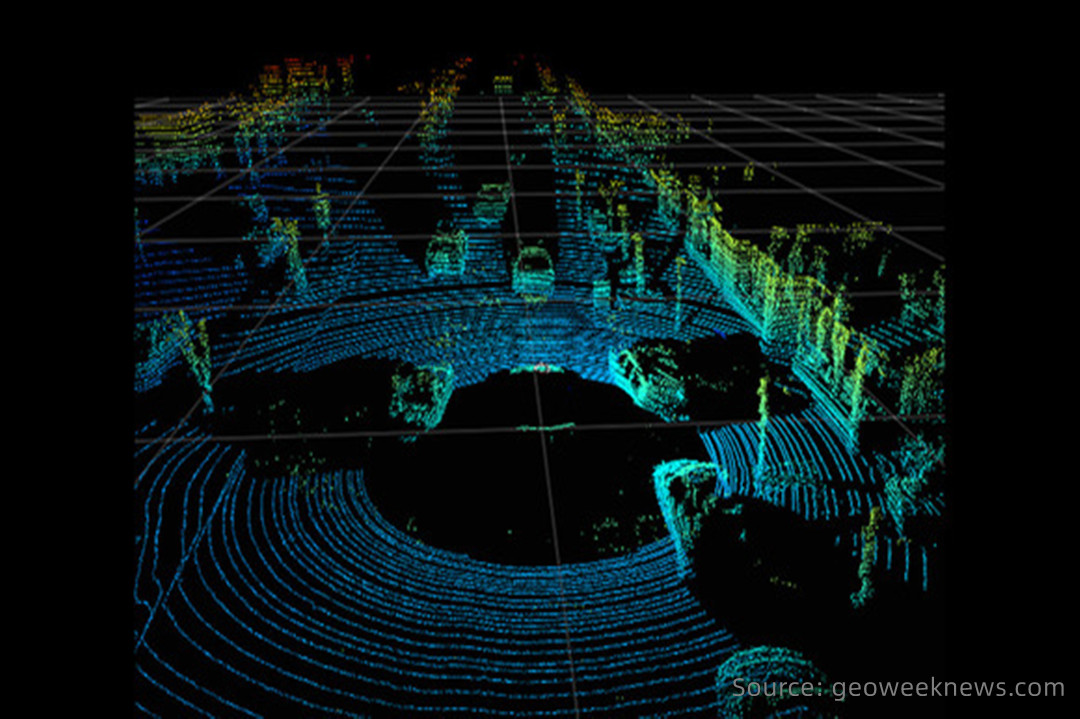
4D LiDAR
4D LiDAR goes beyond the static nature of 3D LiDAR by adding the dimension of time. This enhancement enables the sensor to capture changes and movements in the environment over time. Suited for dynamic environments where tracking moving objects or monitoring changes is crucial, 4D LiDAR finds applications in traffic management, security surveillance, and monitoring the flow of crowds in public spaces. It enhances situational awareness by not only providing a detailed 3D map but also allowing for the tracking of changes over time. This capability enables predictive analysis and monitoring of temporal dynamics.
Based on Modulation Techniques
The modulation technique employed by LiDAR sensors determines how the laser pulses are modulated, impacting factors like range accuracy, resolution, and interference resistance.
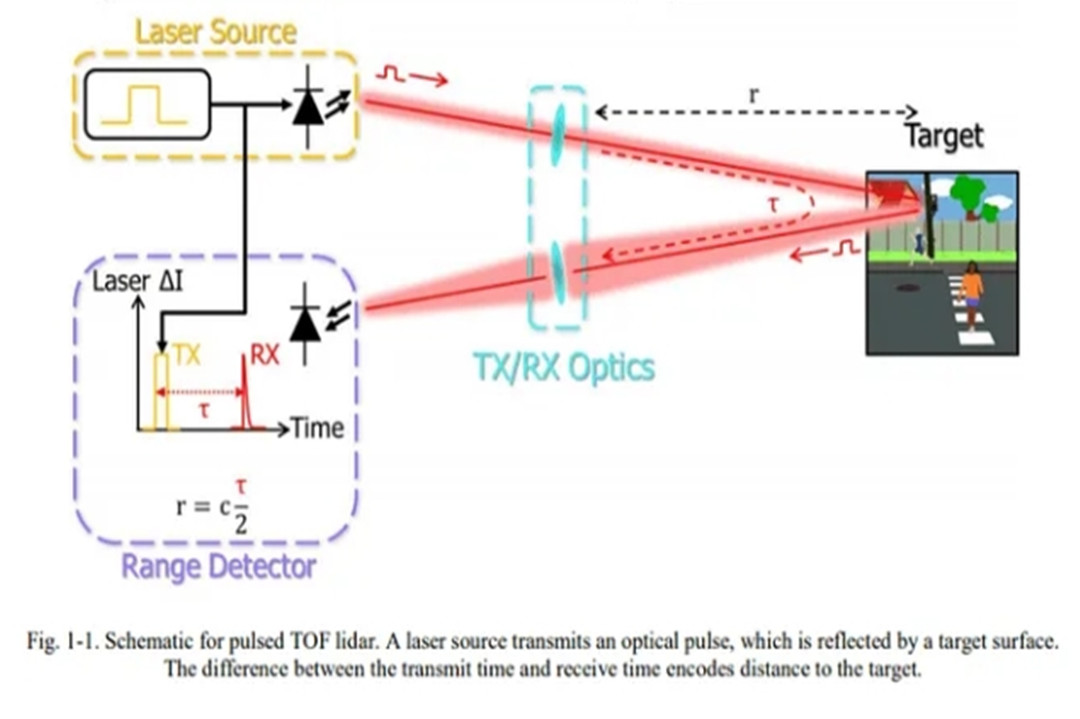
Time-of-Flight (ToF) LiDAR
ToF LiDAR operates based on the principle of measuring the time it takes for a laser pulse to travel to a target and back. This system emits short pulses of laser light and measures the time delay for the reflected light to return. The distance to the object is then calculated using the speed of light. One advantage of ToF LiDAR is its ability to provide accurate distance measurements with high resolution. However, it may face challenges in accurately measuring longer distances and suffers from potential interference issues.
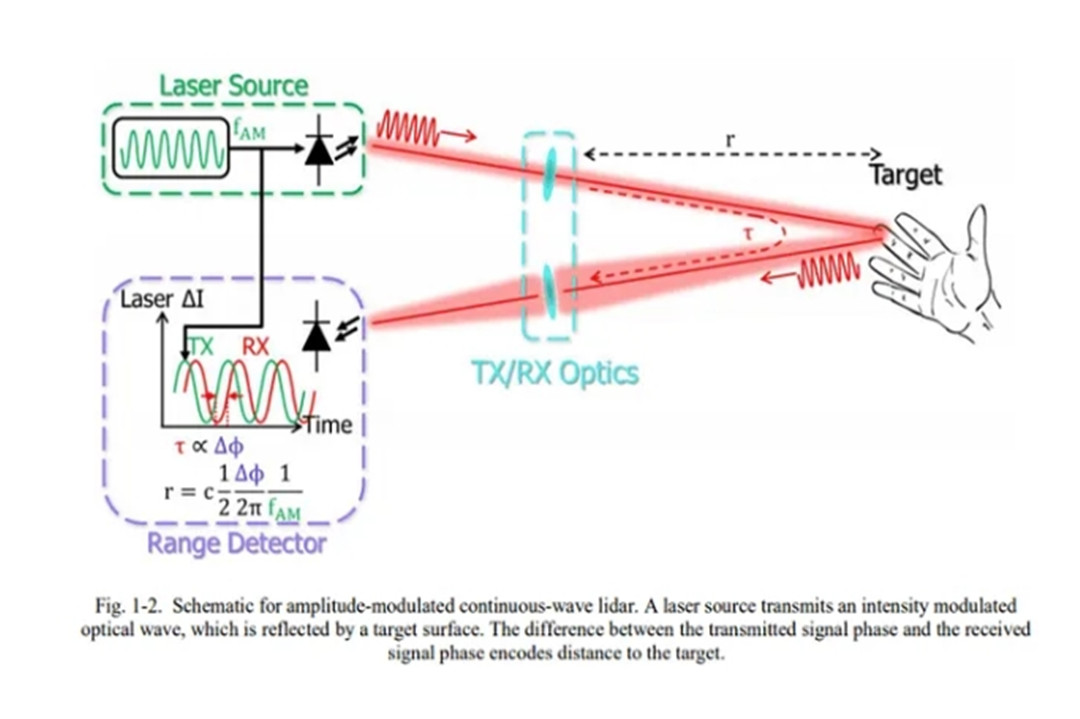
Amplitude Modulated Continuous Wave (AMCW) LiDAR
AMCW LiDAR, on the other hand, utilizes a continuous laser beam with its amplitude modulated at a known frequency. By analyzing the phase shift between the emitted and received signals, the system determines the distance to the target. AMCW LiDAR is known for its capability to measure both distance and velocity accurately. Its advantages include the ability to handle various environmental conditions, but it may have limitations in terms of resolution and susceptibility to interference.
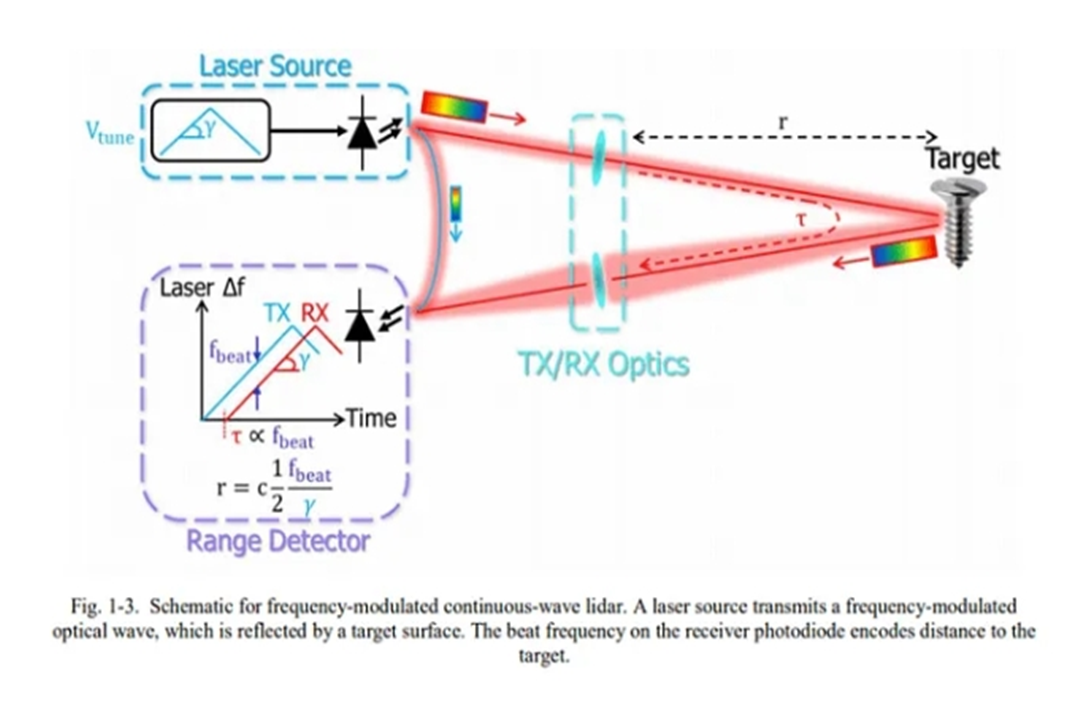
Frequency Modulated Continuous Wave (FMCW) LiDAR
FMCW LiDAR, in contrast, employs a continuous laser beam that is frequency modulated. The distance is calculated by analyzing the frequency difference between the emitted and received signals. FMCW LiDAR offers advantages such as high accuracy and the ability to measure multiple targets simultaneously. However, it may face challenges in terms of complexity and cost.
Based on Primary Measurement Principles
The primary measurement principles of LiDAR sensors define the underlying physics or mathematical concepts governing their operation. Understanding these principles is essential for selecting the right sensor for specific applications.
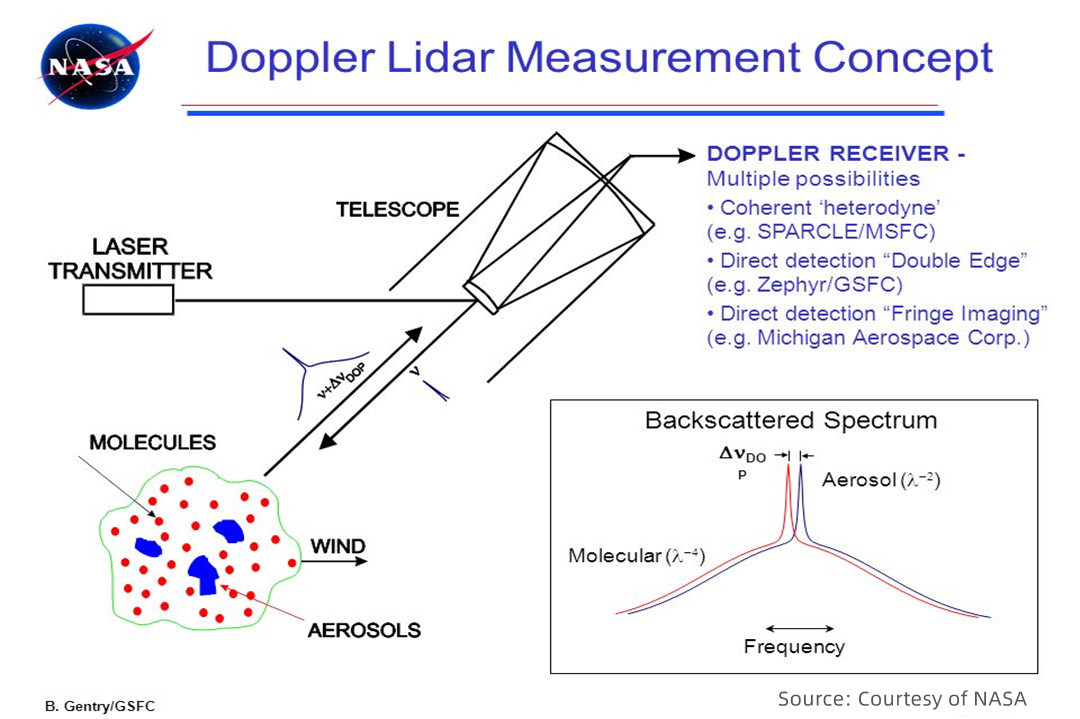
Doppler LiDAR
Doppler LiDAR assesses the velocity of a target by examining the frequency shift of laser light scattered by moving particles in the atmosphere, leveraging the Doppler effect. This frequency change occurs when the target is in motion. Doppler LiDAR is commonly used for measuring wind speed and direction in applications such as atmospheric research and wind resource assessment for wind energy. Its capability to provide velocity information makes it valuable in understanding dynamic atmospheric conditions.
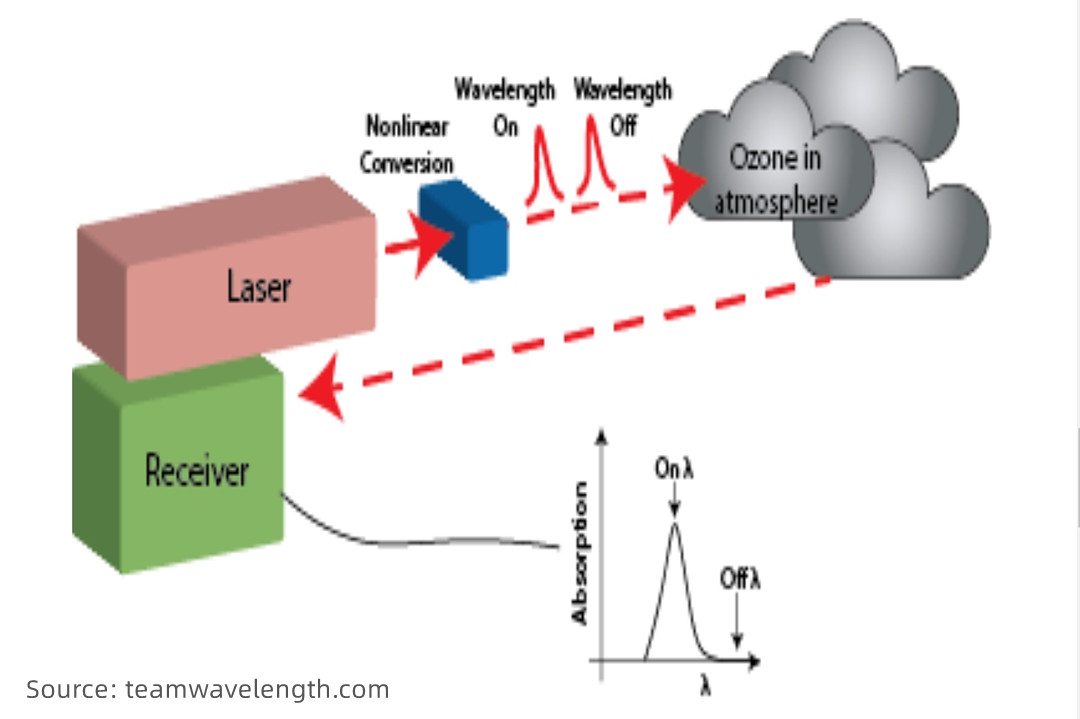
Differential Absorption LiDAR (DIAL)
Differential Absorption LiDAR (DIAL), a specialized Lidar variant, focuses on measuring atmospheric gas concentrations. It utilizes two laser wavelengths - one absorbed by the target gas and another not. By comparing backscattered signals from both wavelengths, DIAL determines the concentration of the specific gas being targeted. This makes DIAL LiDAR crucial for remote sensing of atmospheric composition, commonly employed in monitoring air quality by measuring pollutant concentrations.
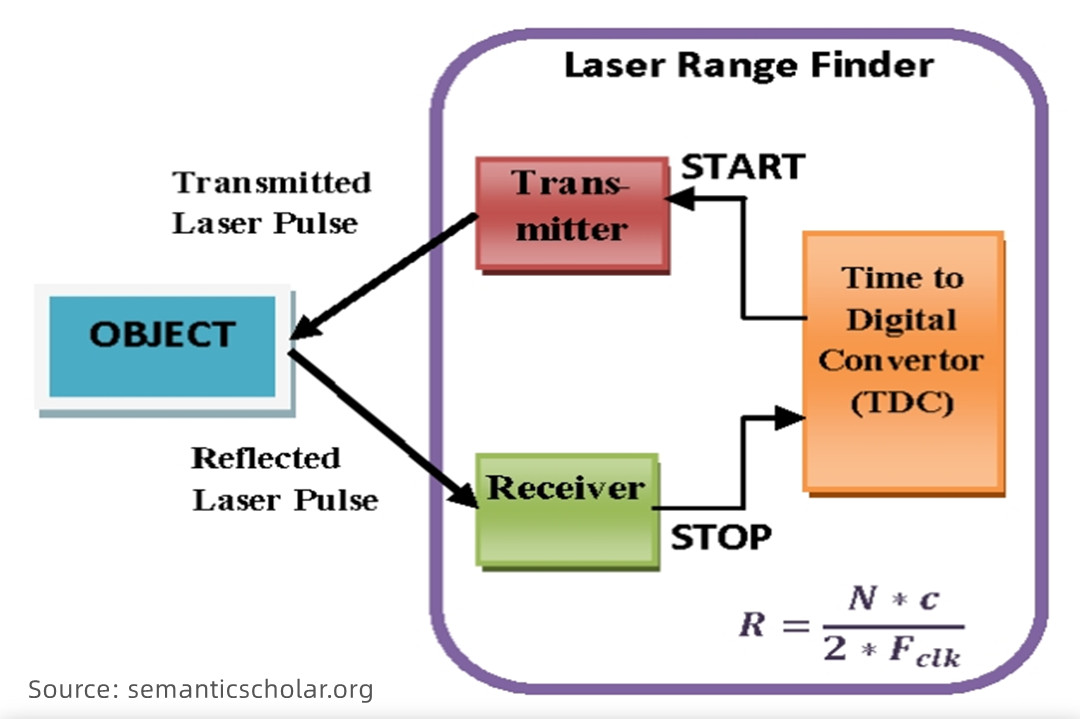
Range Finder
Range Finder LiDAR calculates distances by measuring the time it takes for a laser pulse to travel to a target and back. The distance is determined by multiplying the speed of light by the round-trip time. This type of LiDAR is commonly employed in topographic mapping, forestry, and autonomous vehicles due to its accuracy in distance measurement.
How Does LiDAR Work?
LiDAR, whether deployed on aircraft, drones, or handheld devices, operates on a fascinating principle: shooting a potent laser beam at a target and meticulously measuring its rebound. Think of it as akin to radar and sonar but with light instead of radio or sound waves.
At its core, a LiDAR system consists of three key components: the laser, the scanner, and the Global Positioning System (GPS).
Source: yellowscan.com
1. The Laser
LiDAR systems can employ different types of laser sources, each tailored to specific applications. Continuous wave (CW) sources emit a continuous stream of light, which is commonly used in LiDAR systems for measuring velocity or chemical constituents.
On the other hand, pulsed and chirped sources emit short bursts or pulses of laser light which are particularly useful for precise range measurements in LiDAR systems.
2. The Scanner
Directed by rotating or oscillating mirrors, the laser pulses systematically cover a wide area, scanning the Earth's surface. As these pulses encounter objects, they bounce back toward the LiDAR sensor, creating a comprehensive dataset.
3. Global Positioning System (GPS)
The integration of GPS receivers is paramount for precision. By providing accurate x, y (horizontal), and z (altitude) positions of the LiDAR sensor, GPS ensures the creation of meticulous 3D maps.
The magic of LiDAR unfolds with the laser pulses. Emitting pulses of invisible light, LiDAR maps surfaces by measuring the time it takes for these pulses to return. The formula at play is simple yet powerful:
d = c * t / 2
In the formula, each letter represents:
- d is the distance
- c is the speed of light
- t is the time of the flight
LiDAR doesn't just interact with one surface; a single pulse may encounter multiple surfaces, creating what's known as "first return" (often the topmost object) and subsequent returns, revealing features beneath the initial layer.
To cover extensive areas, LiDAR systems employ scanning mechanisms involving rotating mirrors or similar devices. Meanwhile, precise location data is ensured by integrating multiple GPS or GNSS satellites and receivers, and often, inertial navigation system instruments in aircraft correct for movements induced by air currents.
The culmination of these measurements manifests as a point cloud - a rich 3D representation of the surveyed area. Through meticulous processing and analysis, this data becomes a valuable resource for diverse applications, from urban planning to forestry and the navigation of autonomous vehicles. LiDAR, with its laser precision and technological finesse, paints a vivid picture of our surroundings in three dimensions.
What Are LiDAR Returns?
LiDAR laser returns involve the reflection of laser pulses emitted from a LiDAR system as they interact with various objects on and above the ground surface, such as vegetation, buildings, and bridges. The number of returns for a single emitted laser pulse depends on the number of reflective surfaces encountered during its journey. If a laser pulse encounters multiple reflection surfaces, it is split into as many returns as there are reflective surfaces.
The first returned laser pulse holds significant importance, as it is associated with the highest feature in the landscape, such as a treetop or the top of a building. In some cases, the first return can also represent the ground, resulting in a single return detected by the LiDAR system. Multiple returns, on the other hand, are capable of capturing elevations from several objects within the laser footprint of an outgoing pulse.
Intermediate returns, positioned between the first and last returns, are often utilized for analyzing vegetation structure. In contrast, the last return is typically employed for generating bare-earth terrain models. It's important to note that the last return doesn't always originate from the ground. For instance, if a laser pulse hits a thick branch on its way to the ground and doesn't reach the ground surface, the last return is not from the ground but from the branch that reflects the entire laser pulse.
What Are the Attributes of LiDAR Data?
LiDAR data stands at the forefront of spatial data acquisition, providing precise and detailed information about the Earth's surface. Beyond the fundamental x, y, and z positional values, LiDAR data points are associated with a range of attributes that offer valuable insights into the characteristics of the scanned environment. These attributes, recorded for each laser pulse, significantly enhance the utility of LiDAR data across various applications. Below are key attributes commonly found in LiDAR datasets:
LiDAR Attribute | Description | Application |
---|---|---|
Intensity | Reflects the return strength of the laser pulse, aiding in the identification of surface reflectivity. | Useful for material differentiation and understanding the physical properties of surfaces. |
Return Number | Indicates the order of laser pulse returns, providing insights into reflected features and scanner capabilities. | Facilitates the analysis of multi-return data, contributing to the understanding of complex surface structures. |
Number of Returns | Represents the total number of returns for a given pulse, offering insights into the complexity of scanned features. | Assists in assessing the distribution and behavior of laser returns, particularly in areas with varying surface characteristics. |
Point Classification | Assigns a classification to each LiDAR point, categorizing objects such as ground, vegetation, and water. | Enables detailed land cover analysis, supporting resource management and environmental monitoring. |
Edge of Flight Line | Flags points at the edge of the flight line, with a value of 1 for these points and 0 for others. | Supports quality control and spatial analysis by identifying points located at the boundary of the flight line. |
RGB (Red, Green, Blue) | Provides color information associated with LiDAR points, often derived from concurrent aerial imagery. | Enhances visual interpretation and enables the creation of true-color point clouds for detailed visualization. |
GPS Time | Represents the GPS time stamp at which the laser point was emitted, measured in GPS seconds of the week. | Facilitates temporal synchronization and accurate time-referencing of LiDAR data, crucial for time-sensitive analyses. |
Scan Angle | Specifies the angle at which the laser pulse was emitted concerning the aircraft's orientation, measured in degrees. | Provides insights into the viewing geometry, supporting quality control and analysis of LiDAR data. |
Scan Direction | Indicates the direction of the laser scanning mirror at the time of the output laser pulse, with positive and negative values denoting different directions. | Aids in understanding the scanning pattern and the orientation of the LiDAR sensor during data acquisition. |
What Are Common Types of LiDAR Data Formats?
LiDAR data is stored in various file formats, each catering to specific needs and applications. Here are some common file formats and concepts associated with LiDAR data:
File Format | Description | Attributes | Compression |
---|---|---|---|
LAS | Binary format for LiDAR data. Widely used. | XYZ coordinates, intensity, classifications, metadata | Typically not compressed, but can be compressed using other methods |
LAZ | Compressed version of LAS using LASzip. | Same as LAS, with reduced file sizes | Utilizes LASzip compression, significantly reducing file sizes |
ASCII | Text-based format, human-readable. | XYZ coordinates, intensity, additional attributes | ASCII files are uncompressed and larger in size |
PLY | Polygon File Format for 3D geometric data. | XYZ coordinates, color, custom properties | Can be compressed using various methods |
XYZ | Simple text-based format with XYZ coordinates. | Basic point cloud data, easy to read | Uncompressed text format, larger file sizes |
e57 | Industry-standard for 3D point cloud data. | XYZ coordinates, color, intensity, metadata | Supports compression (e.g., gzip, LZ77), reducing file sizes |
LiDAR Applications
Here's a glimpse into the myriad applications that harness the power of LiDAR technology:
Autonomous Vehicles
LiDAR technology serves as the vigilant eyes of autonomous vehicles, playing a pivotal role in enabling safe and efficient navigation. Here's how LiDAR is harnessed for autonomous driving:
Navigation
LiDAR, with its laser precision, essentially paints a dynamic picture of the world around an autonomous vehicle. As the car cruises, LiDAR continuously sends out laser beams, creating a detailed 3D map – a sort of digital twin of the real world. This real-time mapping is like a guiding hand, helping the car to precisely position itself and navigate through the maze of the urban or suburban landscape.
Think of it as a constantly evolving GPS but on a much more detailed level. It's not just about knowing where the car is; it's about understanding the surroundings down to the centimeter, allowing the vehicle to make split-second decisions based on the most up-to-date information about its environment.
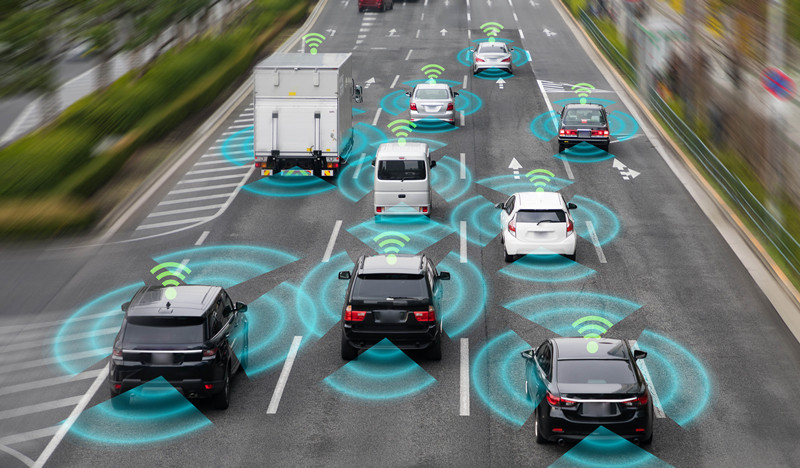
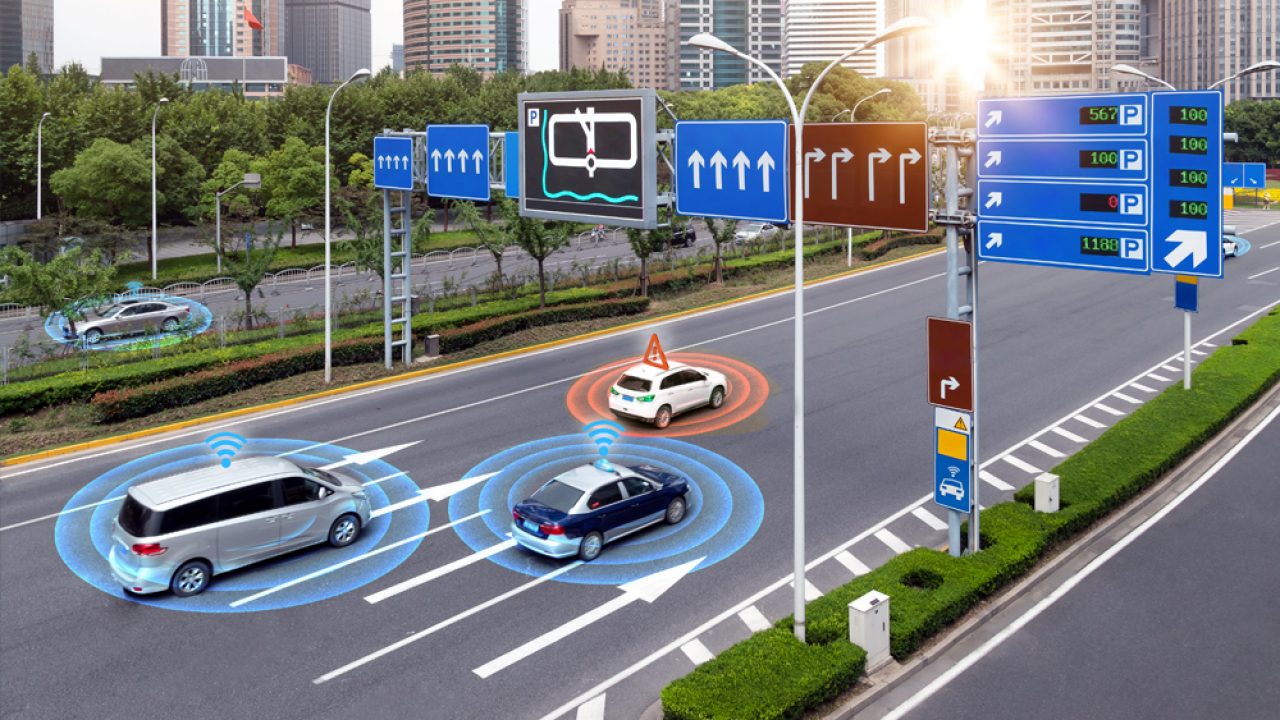
Collision Avoidance
LiDAR is the watchful eye that spots potential collisions before they happen. Picture this: as the car moves, LiDAR beams go out, bouncing off objects and returning with information about what's ahead. If there's a sudden obstacle, like a pedestrian crossing the street or a car making an unexpected turn, LiDAR detects it in real time.
What makes it truly remarkable is its ability to not just see obstacles but to help the car intelligently respond. If there's a potential collision, the autonomous system can swiftly recalculate the route, making split-second decisions to steer clear of danger. It's like having a co-pilot with superhuman reflexes, always ready to hit the brakes or swerve to keep everyone safe.
Autonomous Cruise Control (ACC)
Ever been annoyed by a car tailgating you or wished the car in front would speed up? LiDAR is the technology turning that wish into reality. In autonomous cruise control systems, LiDAR is the vigilant sensor on the lookout for the vehicle's surroundings. It's not just maintaining a set speed; it's maintaining a safe distance from the car in front.
By constantly measuring the distance and relative speed between your car and the one ahead, LiDAR enables the vehicle to be a smart and considerate driver. It's not just about cruising on autopilot; it's about doing it in a way that respects the flow of traffic and keeps everyone at a safe distance.
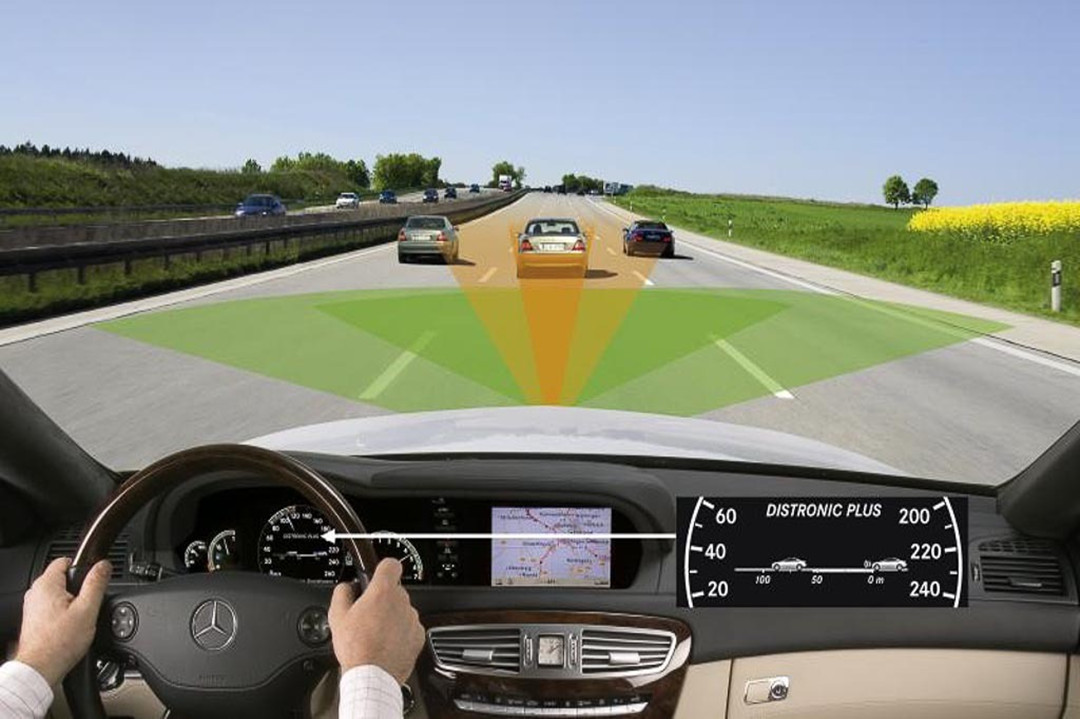
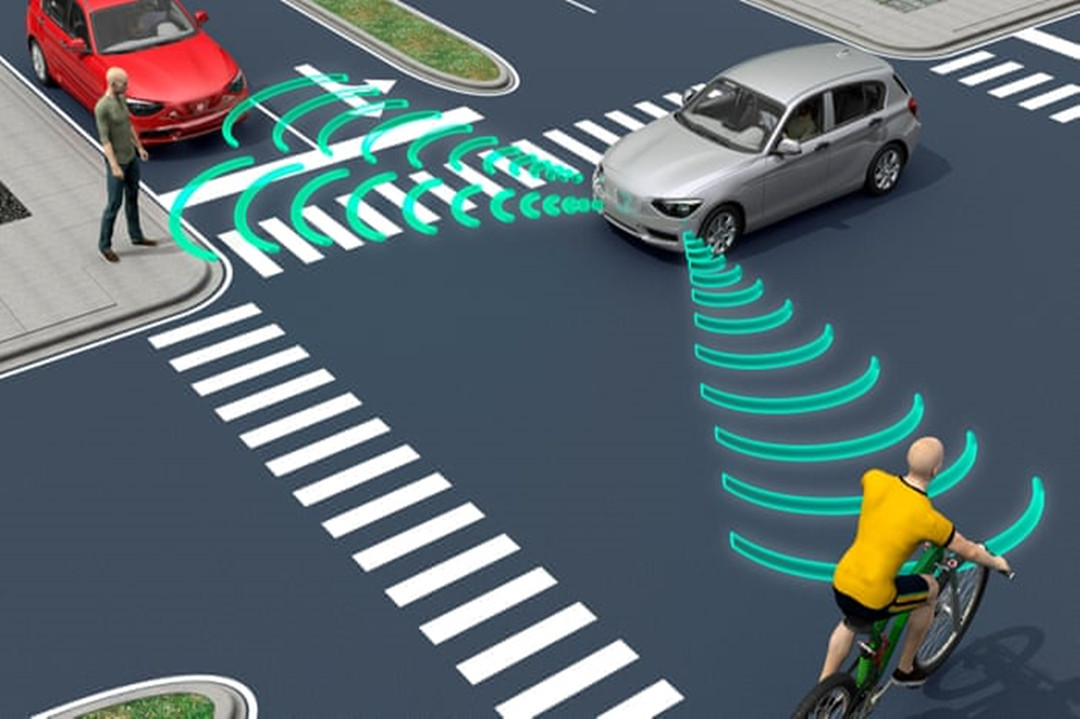
Obstacle Detection
LiDAR turns an autonomous vehicle into a keen observer of its environment. Imagine LiDAR as the eyes of the car, able to see in all directions simultaneously. It doesn't just see objects; it sees them with incredible accuracy, down to the centimeter.
This 360-degree visibility is a game-changer. It means the car not only spots stationary obstacles like lampposts or parked cars but also detects dynamic ones – the pedestrian stepping off the curb or the cyclist coming from the side. And here's the kicker – it does this in all weather conditions. Rain, fog, or sunshine, LiDAR remains a reliable set of eyes, ensuring the car is always aware of what's happening around it.
Aerial Inspection
LiDAR technology transforms aerial inspections by mounting it on drones. like the JOUAV CW-15 with JoLiDAR-LR22S. In a two-hour flight, it covered 52 km, producing geo-referenced point clouds with a remarkable 3 to 6 cm accuracy.
Bridge Inspections
Consider bridge inspections, where LiDAR-equipped drones swiftly and precisely evaluate conditions. They identify potential problems such as corrosion or structural deformations, enabling engineers to prioritize maintenance effectively and enhance overall safety.
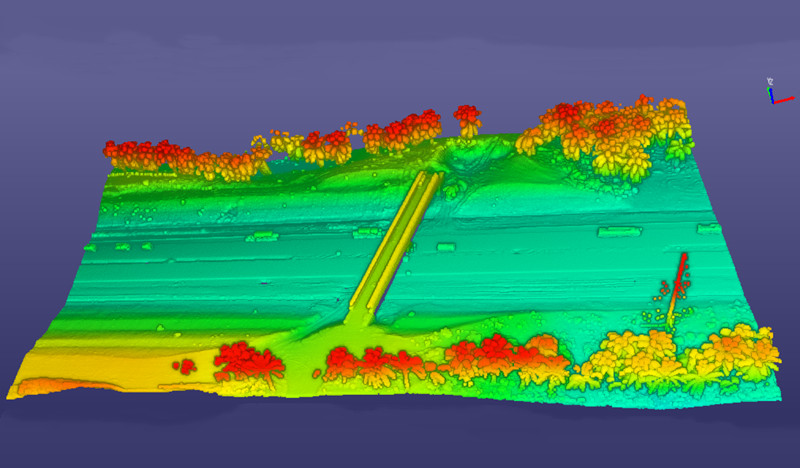
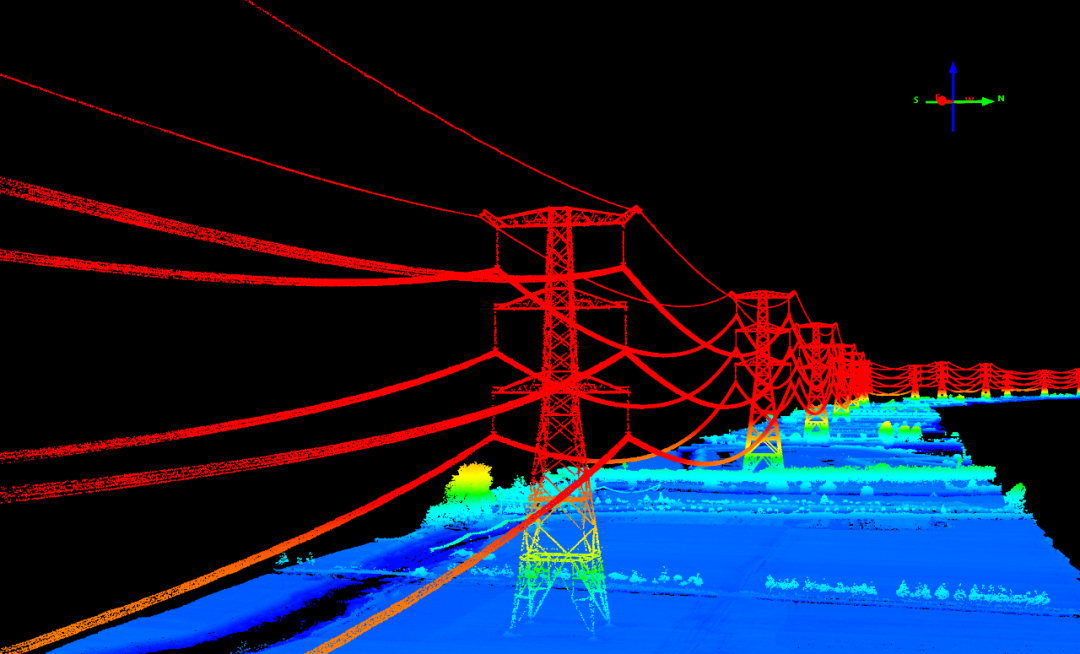
Power Line and Utility Inspections
Power line and utility inspections benefit from LiDAR's detailed data. Drones equipped with LiDAR identify hazards like vegetation encroachment or damaged equipment, allowing for preventive maintenance and ensuring the reliability of energy infrastructure.
Dam and Levee Monitoring
For dam and levee monitoring, LiDAR aids in assessing stability and integrity. By identifying potential issues early on, engineers can take timely steps to address concerns, ensuring the ongoing safety of critical water management systems.
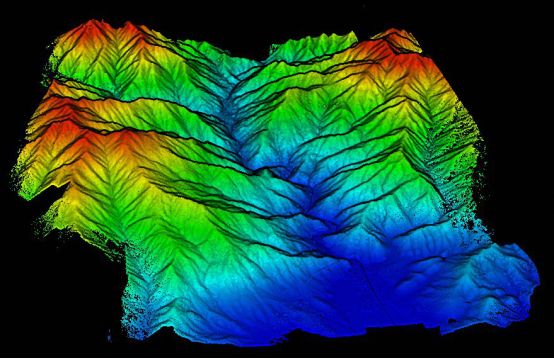
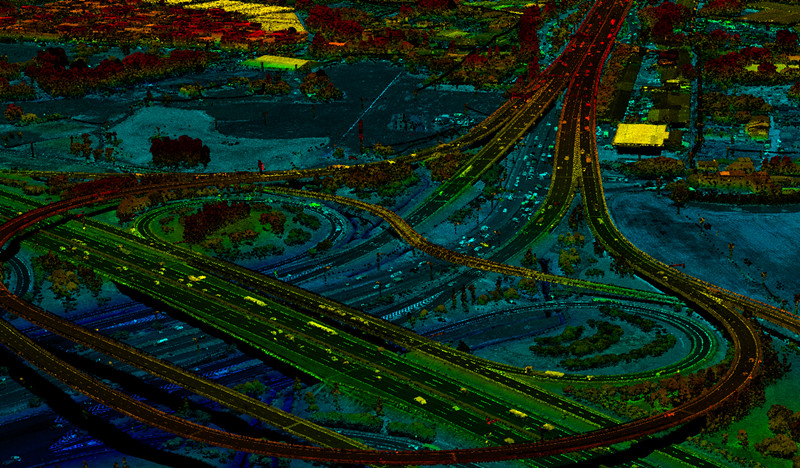
Railway and Transportation Systems
Railway and transportation systems also leverage LiDAR. Drones inspect tracks, tunnels, and infrastructure, detecting issues such as deformations or obstructions. This proactive approach minimizes disruptions and maintains the safety and efficiency of transportation systems.
Precision Agriculture
LiDAR data proves invaluable in forecasting crop yields and monitoring crop conditions throughout the growing season. This analytical advantage enables farmers to predict yields more accurately, facilitating informed decisions to maximize agricultural income.
Soil Insight and Analysis
Utilizing geospatial data from LiDAR for digital soil mapping enhances soil insight. It delves into topsoil quality, soil classification, and drainage efficiency. This knowledge is vital for optimizing irrigation strategies and avoiding waterlogging in specific areas.
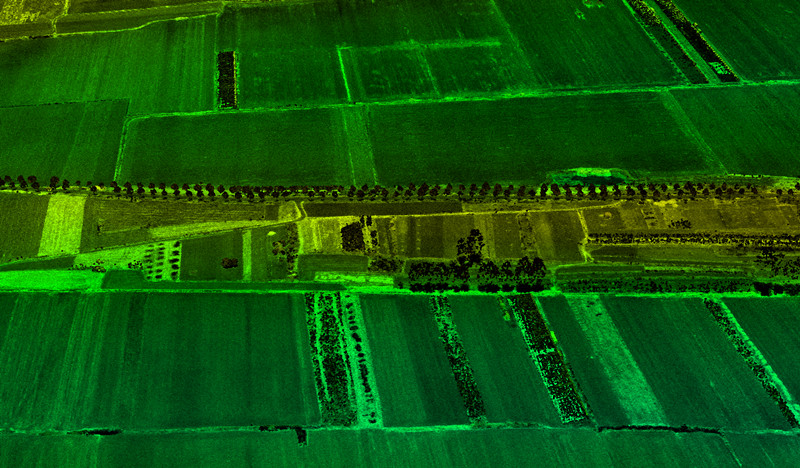
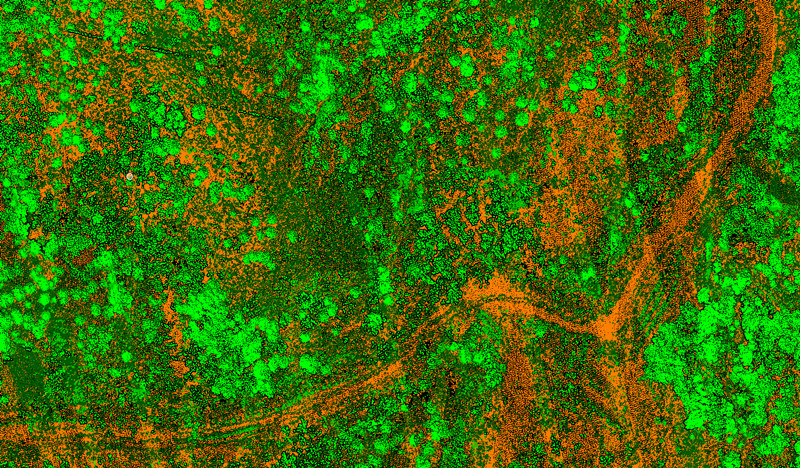
Improving Irrigation
Efficient irrigation planning becomes possible by identifying water sources and mapping out water distribution. LiDAR aids in steering clear of sections prone to water pooling or where soils retain moisture more effectively, ensuring judicious use of water resources.
Minimizing Erosion
Understanding the slope of your land enables erosion minimization. Even minor shifts in elevation, when detected in advance, allow for proactive measures to prevent soil erosion, safeguarding valuable topsoil and sustaining crop health.
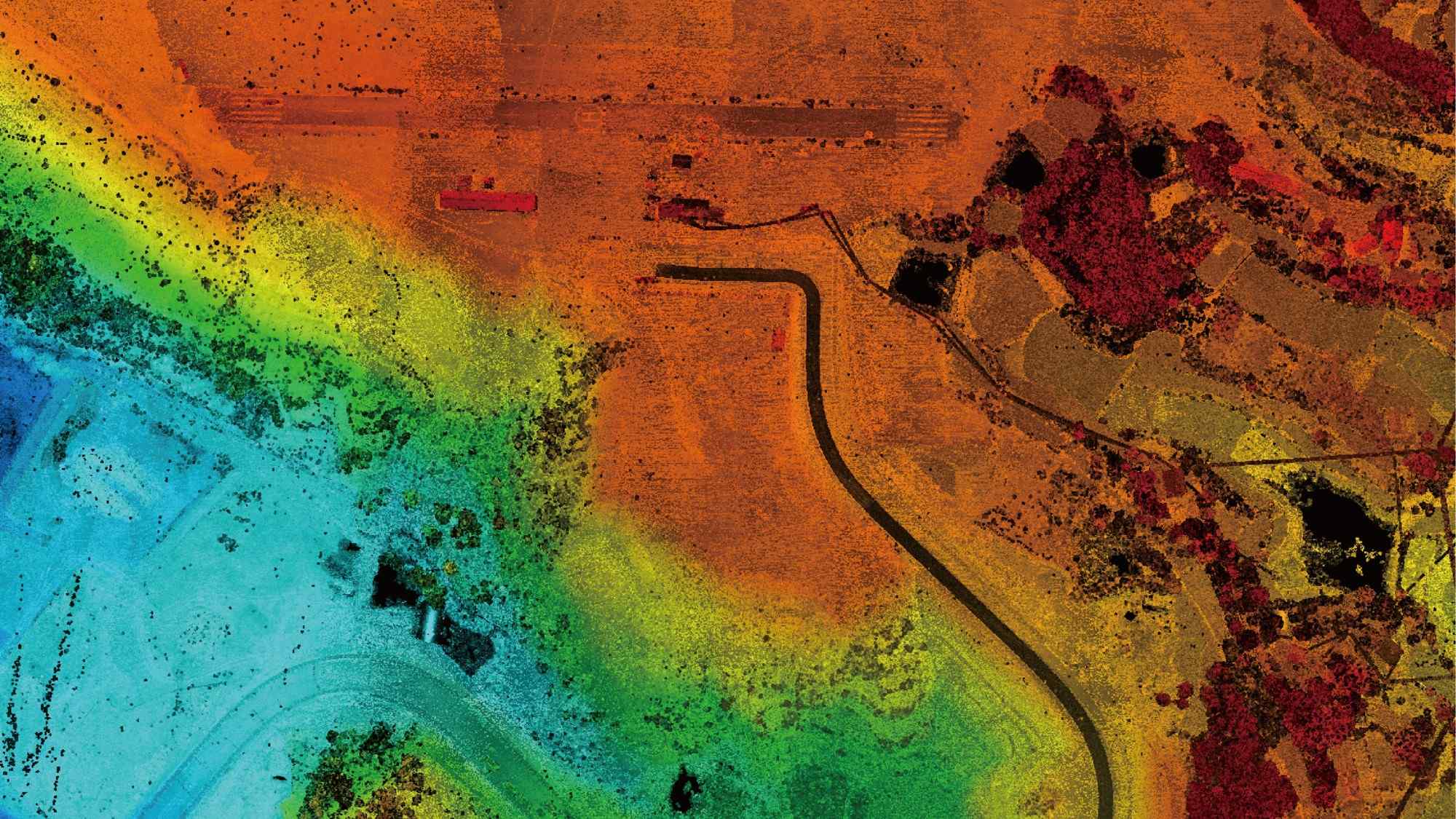
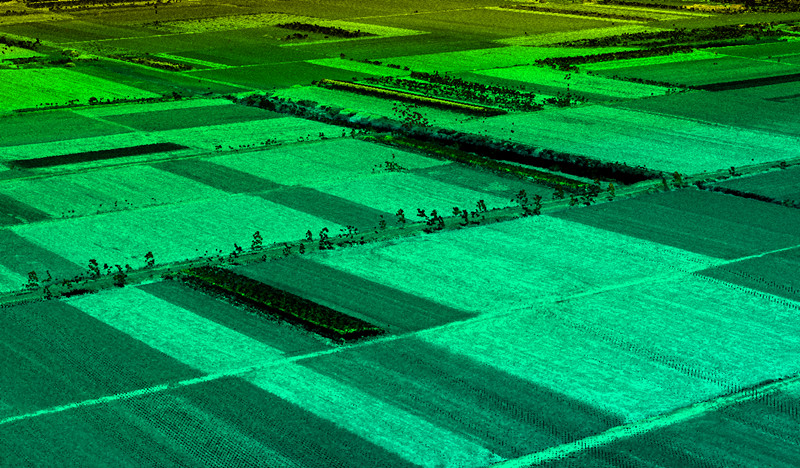
Planning Crop Planting
In large-scale farming, variations in sun exposure, water drainage, and erosion necessitate meticulous planning. LiDAR-derived insights empower farmers to strategically plan crop planting, optimize output and minimize losses based on the diverse conditions across the farm.
Forecasting Crop Yields and Output
LiDAR data proves invaluable in forecasting crop yields and monitoring crop conditions throughout the growing season. This analytical advantage enables farmers to predict yields more accurately, facilitating informed decisions to maximize agricultural income.
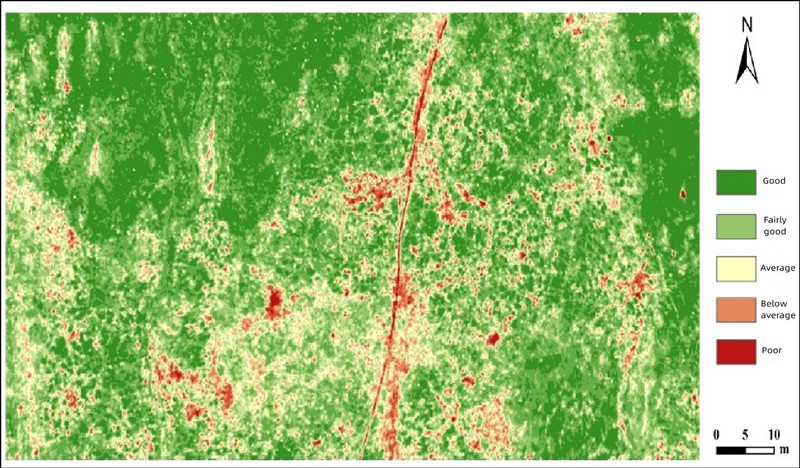
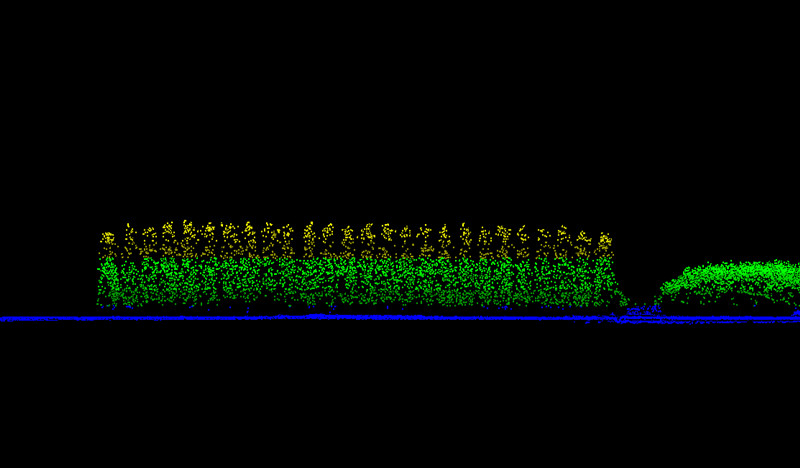
Determining Crop Damage
In the event of severe weather events or other issues affecting crops, LiDAR provides an accurate assessment of damage severity. Unlike traditional monitoring methods, LiDAR offers a detailed and comprehensive view of the impact, aiding farmers in assessing losses and planning recovery strategies effectively.
Forestry Management
LiDAR technology plays a multifaceted role in forestry management, offering comprehensive solutions for various aspects of environmental monitoring and conservation.
Controlling Deforestation
Aerial LiDAR swiftly evaluates the extent of deforestation by assessing the volume of felled trees. It enables specialists to compare actual volumes with predicted ones, facilitating the identification of inconsistencies and aiding in efforts to control deforestation.
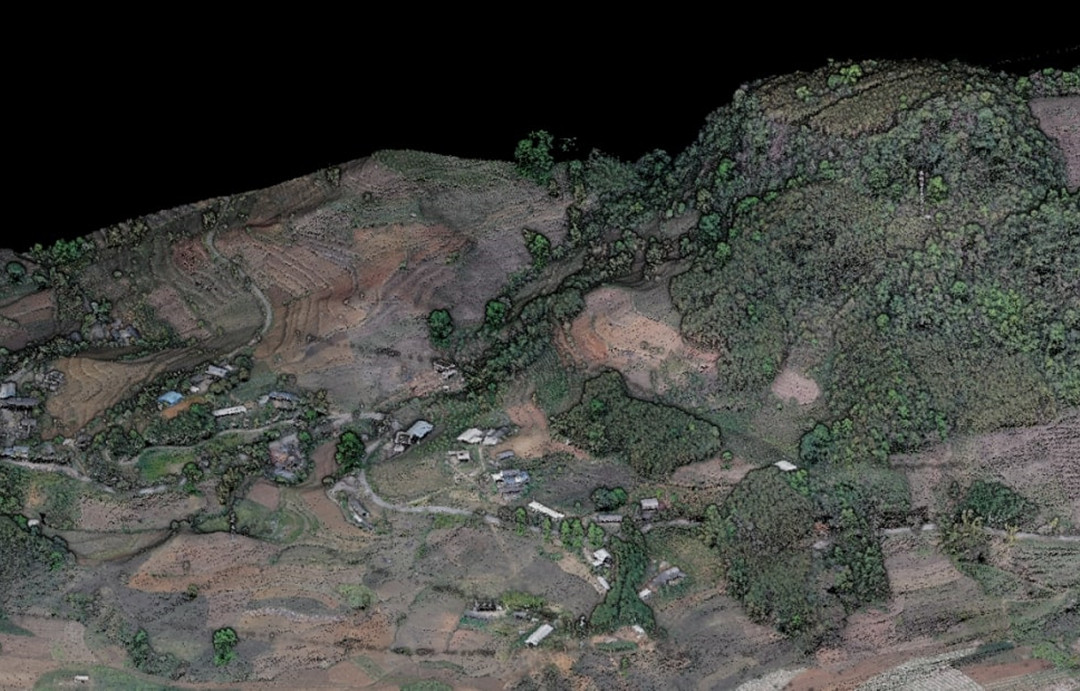
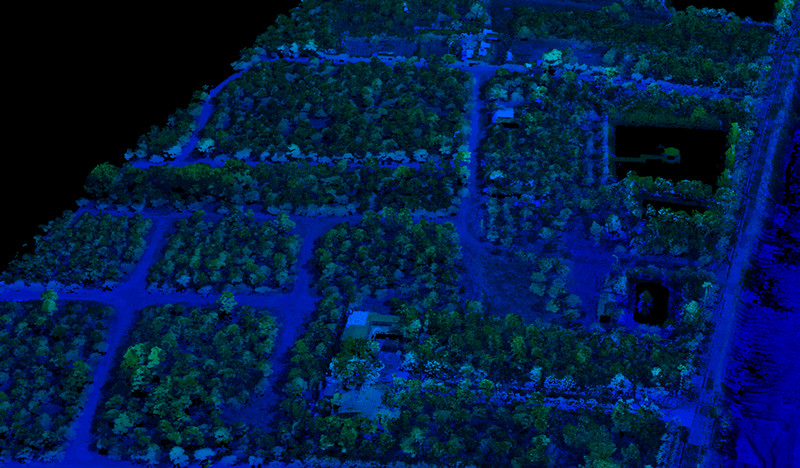
Forest Planning and Management
Utilizing LiDAR drones facilitates powerline scanning to detect potential clashes with tree canopies. It contributes to overall forest planning by mapping digital elevation, assisting in topography assessments, and documenting intricate forest environments.
Identifying Tree Stem Attributes
LiDAR captures detailed data, and specialized algorithms are employed to detect individual trees and identify their positions. These algorithms segment trees, enabling efficient tree counts over large areas, a valuable tool for effective forest management.
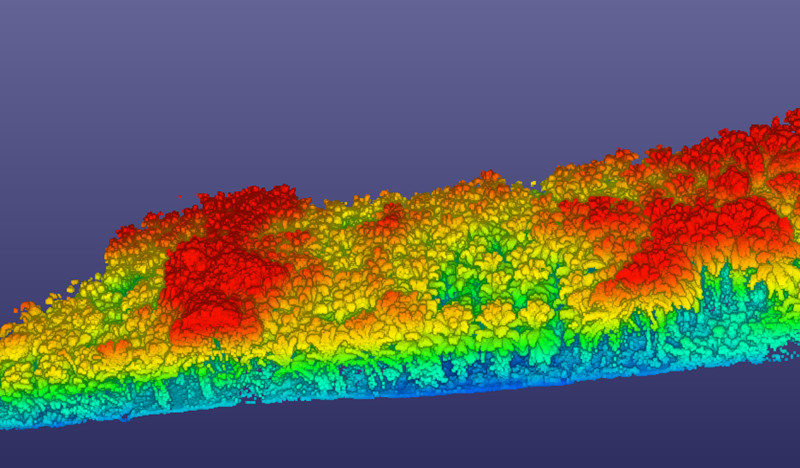
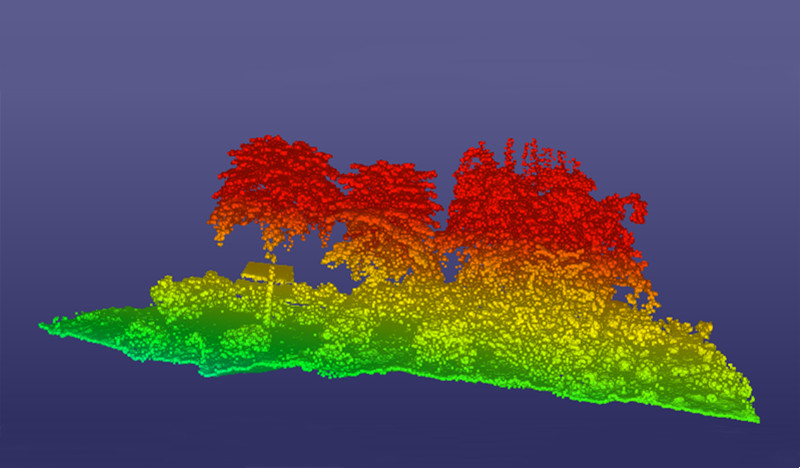
Measuring Carbon Absorption
LiDAR data analysis is instrumental in determining carbon absorption rates. By examining individual stand attributes, such as tree or crown diameter, scientists gain insights into the carbon absorption properties of the forest, contributing to ecological understanding and carbon accounting.
Wildlife Conservation
Post-processing analysis of LiDAR data provides valuable measurements, including biomass and densitometric data, supporting assessments of forest suitability for specific wildlife species. This aids in strategic forest planning and conservation efforts, ensuring ecosystems are conducive to various forms of wildlife.
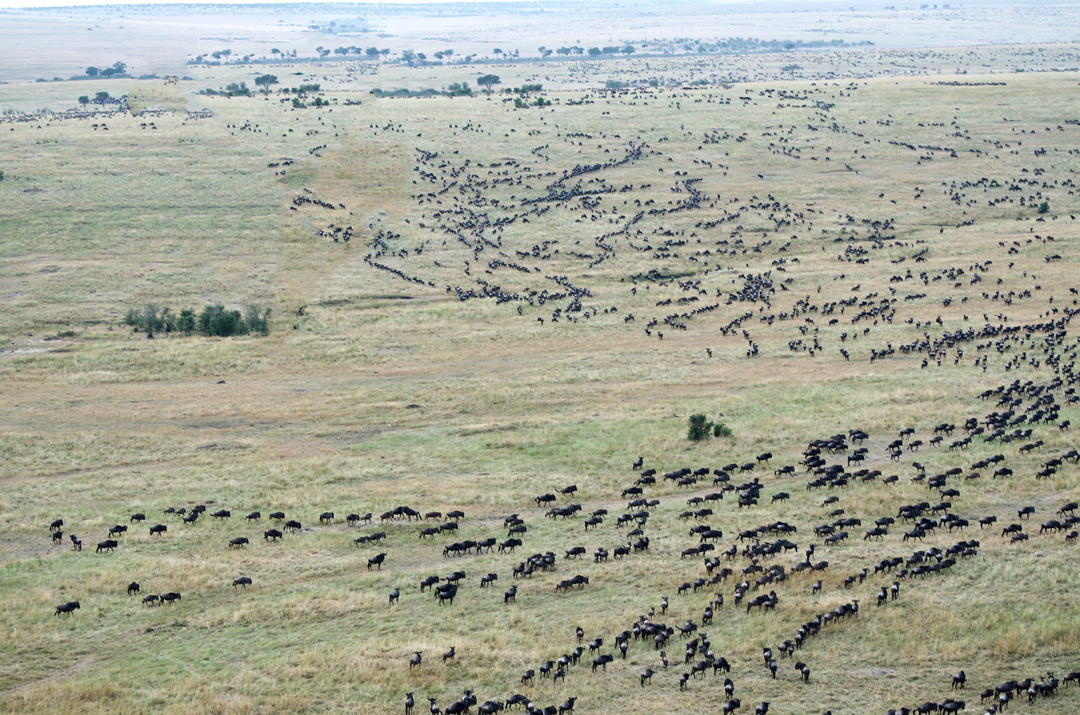
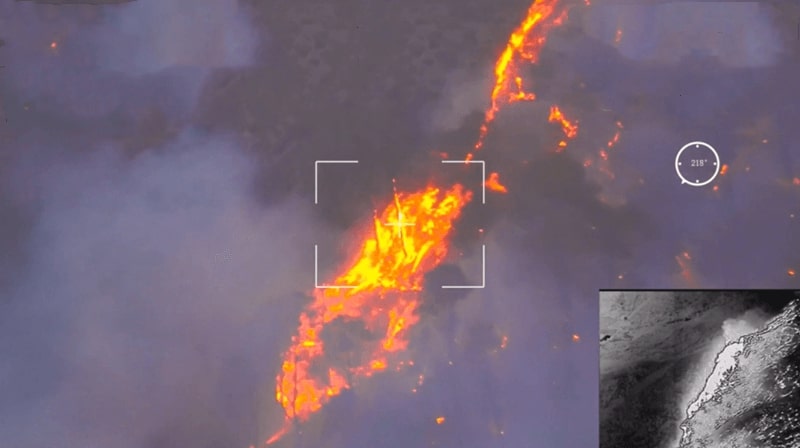
Forest Fire Management
In the context of climate change mitigation, LiDAR technology is employed to predict and monitor forest fire risks. LiDAR data identifies wood debris on the ground, serving as potential fire tinder. This information is crucial for tracking wildfire patterns and understanding historical fire paths, aiding in effective forest fire management strategies.
Robotics
LiDAR technology is a game-changer for robotics, bringing precision and adaptability to various crucial functions:
Navigation
Navigational prowess is heightened as LiDAR sensors furnish real-time insights into the robot's surroundings. Through emitting laser beams, LiDAR crafts a dynamic 3D map, empowering robots to chart their course autonomously.
Obstacle Avoidance
Obstacle avoidance becomes seamless with LiDAR, enabling robots to swiftly detect and sidestep barriers like walls and doors. This real-time obstacle detection is pivotal for ensuring safe and efficient robot movement in ever-changing environments.
Localization
Accurate localization is a forte of LiDAR. By cross-referencing real-time sensor data with existing maps, robots pinpoint their exact location with precision, elevating the reliability of their navigation.
Mapping
Mapping capabilities reach new heights as LiDAR's laser beams meticulously measure distances in three dimensions. This detailed mapping equips robots with a comprehensive understanding of their environment, aiding decision-making processes.
Perception
Perception takes center stage as LiDAR sensors provide intricate details about the surroundings. Robots leverage this information to discern objects and people, enabling them to make nuanced decisions and adapt to diverse scenarios.
Real-time Environment Monitoring
The real-time monitoring prowess of LiDAR is indispensable. Continuously observing the environment, LiDAR sensors offer crucial data about dynamic changes, ensuring that robots can flexibly adapt to evolving conditions and navigate with confidence.
Climate Monitoring
LiDAR technology is a linchpin in climate monitoring, seamlessly integrating into various facets of environmental research:
Carbon Sequestration
LiDAR's laser beams penetrate forest canopies, measuring the vertical structure of vegetation. This information assists in estimating biomass and assessing forest health. Researchers can use these measurements to understand carbon sequestration capacity, helping in the development of effective strategies for carbon management and climate change mitigation.
Glacier Monitoring
LiDAR plays a crucial role in monitoring changes in glacier volume and extent. By comparing successive LiDAR scans over time, scientists can precisely measure glacial retreat or advancement, contributing valuable data for understanding the impact of climate change on glacial systems and predicting potential sea-level rise.
Coastal Zone Management
LiDAR assists in studying coastal dynamics by monitoring shoreline changes and erosion. This information is essential for coastal zone management and understanding the vulnerability of coastal areas to rising sea levels and extreme weather events.
Air Quality Monitoring
LiDAR, when integrated with other sensors, provides high-resolution data for monitoring air quality. It can detect and quantify pollutants such as particulate matter, ozone, and gases. This information contributes to assessing the impact of air pollution on climate and human health, supporting the development of effective pollution control measures.
Archaeology
LiDAR technology has revolutionized archaeology by providing detailed and accurate data for mapping and understanding archaeological landscapes.
Site Discovery and Mapping
LiDAR captures high-resolution data that allows archaeologists to identify subtle features on the landscape, such as ancient roads, walls, and foundations. The precision of LiDAR aids in mapping extensive areas efficiently, revealing potential archaeological sites that might be missed through traditional survey methods.
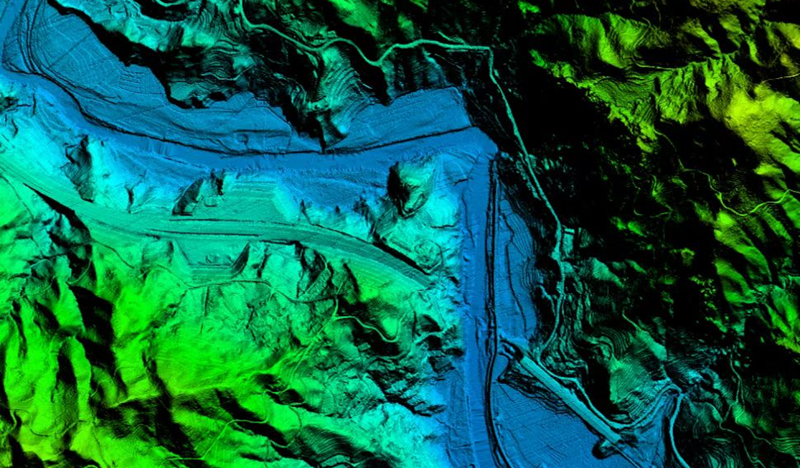
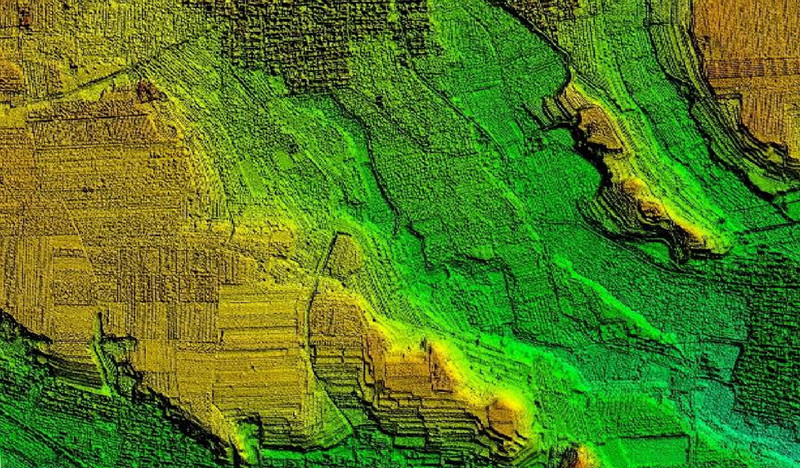
Topographic Modeling
LiDAR creates accurate topographic models, offering a detailed representation of the land surface. This is particularly valuable for studying archaeological sites in varying terrains, enabling researchers to analyze elevation changes and understand how ancient communities interacted with their surroundings.
Identification of Anthropogenic Features
LiDAR aids in the identification of anthropogenic features such as earthworks, mounds, and terraces. By detecting subtle changes in elevation, archaeologists can pinpoint these features, contributing to a more comprehensive understanding of past human activities.
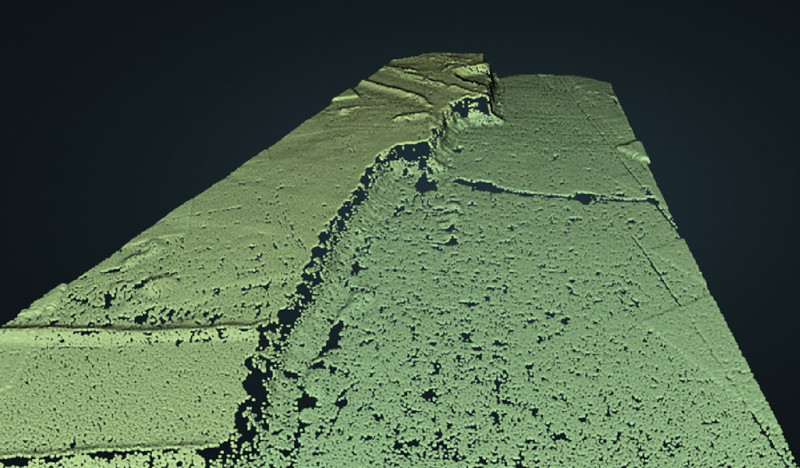
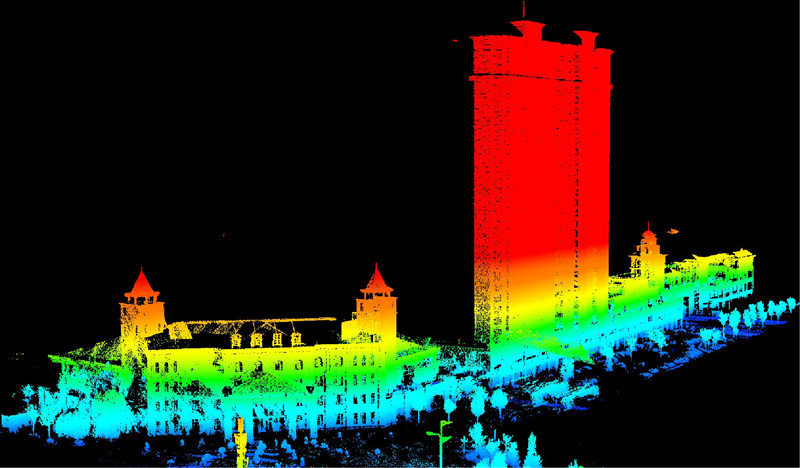
Landscape Analysis
LiDAR facilitates the analysis of settlement patterns by providing a broader view of the landscape. Researchers can study the layout of ancient civilizations, including the distribution of settlements, agricultural areas, and resource usage, contributing to a holistic understanding of past societies.
Cultural Resource Management
LiDAR assists in cultural resource management by providing critical information for assessing the impact of development projects on archaeological sites. This proactive planning ensures the preservation of cultural heritage amid urbanization and infrastructure development.
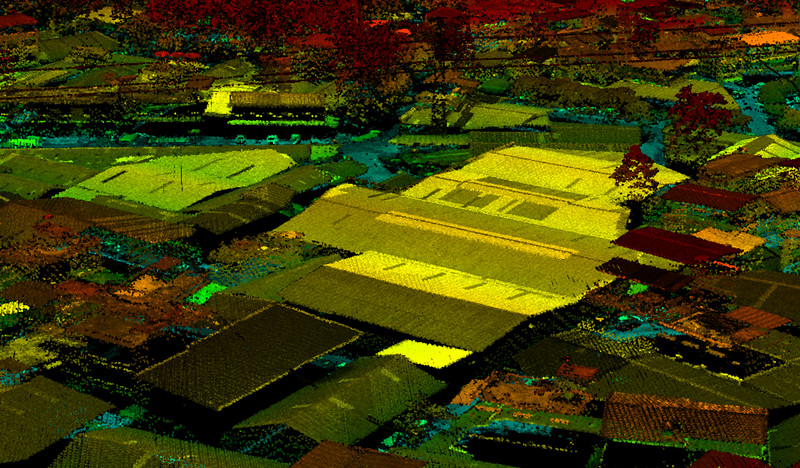
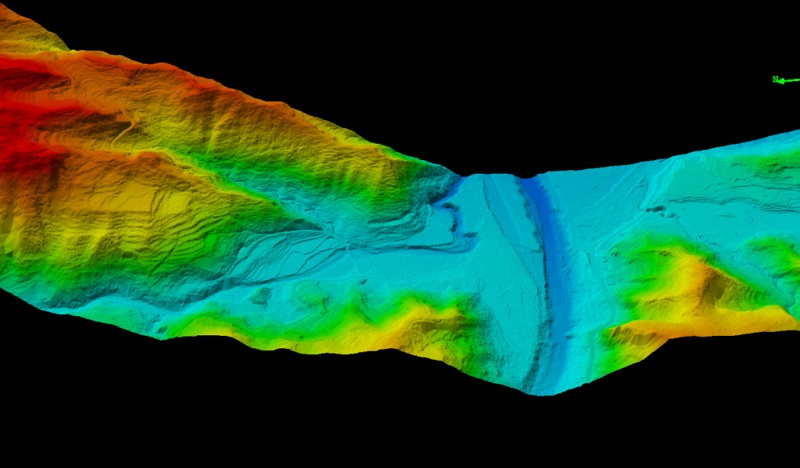
Vegetation Penetration
In areas covered by dense vegetation, LiDAR's ability to penetrate through the canopy unveils archaeological features that might be hidden. This is especially useful in tropical forests, where vegetation can make ground-based surveys challenging.
Police and Law Enforcement
Police use LiDAR technology primarily for traffic enforcement and speed detection. Here's how police use LiDAR devices:
Speed Detection
Police use a LiDAR (laser) gun to measure a vehicle's speed. LIDAR uses a short burst of infrared laser light to reflect off a vehicle and back to the gun. By analyzing the time it takes for the laser beam to travel to the vehicle and back, the LIDAR gun can calculate the speed of the vehicle with high precision.
Distance Measurement
LiDAR technology is employed to measure the distance between vehicles, aiding in enforcing regulations related to safe following distances. This is particularly important for preventing accidents caused by tailgating.
Traffic Flow Analysis
LiDAR devices can be strategically positioned to monitor traffic flow on roads and highways. The data collected helps law enforcement agencies understand traffic patterns, identify congestion points, and optimize traffic management strategies for improved safety and efficiency.
Accident Reconstruction
In the aftermath of a traffic accident, LiDAR technology is used for forensic analysis and accident reconstruction. LiDAR captures detailed information about the accident scene, including the positions of vehicles, road conditions, and other environmental factors. This aids in determining the sequence of events leading to the accident.
What is LiDAR Mapping?
LiDAR mapping employs a laser scanning system equipped with an Inertial Measurement Unit (IMU) and a Global Navigation Satellite System (GNSS) receiver. The simultaneous use of the Simultaneous Localization and Mapping (SLAM) algorithm and Real-Time Kinematic (RTK) technology ensures precise georeferencing of each measurement point in the resulting point cloud.
LiDAR's laser beams create a 3D representation of the target object or area. Each point in the point cloud corresponds to a precise location, allowing for an accurate and detailed spatial model.
LiDAR maps provide both absolute and relative positional accuracy. This means that not only can the viewer determine the geographical location of the data collected, but they can also understand the spatial relationships between points in terms of distance. This dual accuracy is crucial for various applications, from infrastructure planning to environmental monitoring.
What is SLAM? Why Use it For LiDAR Mapping?
SLAM, or Simultaneous Localization and Mapping, is a technology integral to surveying, particularly when coupled with LiDAR. It involves devices that use sensor data to construct a detailed map of their surroundings while simultaneously determining their own position within that environment. This process is vital for various applications, including surveying and mapping.
In the context of SLAM, sensors play a crucial role, gathering data from different sources such as visual data from cameras or non-visible data from Sonar, Radar, or LiDAR. Additionally, basic positional data from an inertial measurement unit (IMU) is utilized. The SLAM device then processes this information to compute the best estimate of its position within the environment.
The iterative nature of SLAM is noteworthy. As the device moves within the environment, all features, such as walls and pillars, change in relation to the device. The SLAM algorithm continually refines its estimate by incorporating new positional information, making it more accurate with each iteration.
In practical terms, a SLAM LiDAR scanner allows for a streamlined surveying process. Users can simply walk through an environment, and the scanner builds a digital map in real-time. This approach eliminates the need for laborious set-ups, resulting in significant time and cost savings. SLAM-based mobile mapping systems are remarkably efficient, offering survey times that are over 10 times faster than traditional methods. Importantly, SLAM systems don't rely on GPS, enabling scanning by hand or by attaching a scanner to various platforms like trolleys, drones, poles, or cradles. The versatility and efficiency of SLAM make it a valuable technology in modern surveying practices.
What is LiDAR Resolution?
LiDAR data resolution refers to the level of detail or granularity present in the acquired point cloud. It essentially describes how finely the laser beams sample the surface of the Earth, resulting in a more or less detailed representation of the environment. The resolution of LiDAR data is a critical factor in various applications where detailed and accurate information about the terrain or objects is essential.
Check out this YouTube video that showcases the resolution of data results gathered at various flight altitudes using different JOUAV UAV models. These drones are equipped with LiDAR sensors boasting different ranges, providing a comprehensive view of the collected data:
Here are some typical resolutions of LiDAR data:
- Low Resolution:
- Less than 2 points per square meter.
- Use Cases: Broad-scale terrain modeling, and large-area mapping where fine details are not critical.
- Medium Resolution:
- Point Density: Between 2 and 10 points per square meter.
- Use Cases: Urban planning, forestry, environmental monitoring, and medium-scale topographic mapping.
- High Resolution:
- Point Density: Between 10 and 20 points per square meter.
- Use Cases: Detailed topographic mapping, infrastructure planning, and applications requiring fine-scale features.
- Very High Resolution:
- Point Density: More than 20 points per square meter.
- Use Cases: Detailed engineering surveys, archaeological studies, and applications requiring a high level of precision.
Choosing the right resolution depends on what you're trying to achieve. Higher resolutions give you more detail, but they also mean bigger data files and more processing work. It's a bit of a balancing act based on what you need for your specific project or application.
Key Factors Affecting LiDAR Resolution:
Pulse Density (Point Density)
Pulse density, also known as point density, is a fundamental factor influencing LiDAR data resolution. It is defined as the number of LiDAR points collected per unit area on the Earth's surface. The higher the pulse density, the more densely sampled the terrain, and consequently, the higher the resolution of the resulting point cloud. High pulse density is particularly important for applications that require a detailed representation of the landscape, such as forestry management or urban planning.
Scanning Density
The scanning density refers to the spatial distribution of laser pulses across the area being surveyed. It is determined by the scanning pattern and frequency of the LiDAR sensor. A LiDAR sensor that emits laser pulses more frequently and in a closely spaced pattern can achieve higher scanning density, leading to increased data resolution. This is crucial for capturing intricate features and details on the ground, especially in complex terrains.
Sensor Characteristics
The specifications of the LiDAR sensor itself significantly impact the achievable data resolution. Key sensor characteristics include the laser beam divergence and the scanning mechanism. A LiDAR sensor with a narrow laser beam can focus on smaller areas with higher precision, contributing to higher resolution. Additionally, a precise scanning mechanism that accurately captures the position and orientation of each laser pulse enhances the overall data resolution.
What is LiDAR Accuracy?
LiDAR accuracy, essentially how closely the collected data aligns with the real-world values, is crucial for its various applications. We often gauge accuracy by calculating the Root Mean Square Error (RMSE), which gives us a measure of the discrepancies between the LiDAR data and the ground truth.
Typically, LiDAR sensors are pretty impressive – they can be accurate within 0.5 to 10mm compared to the sensor itself. When it comes to mapping, they can nail it with up to 1cm accuracy in horizontal (x, y) and 2cm in vertical (z). So, basically, they're really good at capturing details with a high level of precision!
The JOUAV CW-25E VTOL drone equipped with JoLiDAR-1500 acquired vertical accuracy as low as 0.6cm and horizontal accuracy as low as 0.5cm at an altitude of 310m.
There are two main types of LiDAR accuracy: absolute and relative.
Absolute Accuracy
Absolute accuracy involves both horizontal and vertical dimensions. Horizontal accuracy is determined by comparing LiDAR data with ground-surveyed checkpoints, which are well-defined points on the ground with accurately surveyed horizontal positions. Vertical accuracy, on the other hand, is assessed by comparing LiDAR data with surveyed points on flat and open terrain, minimizing interpolation errors. Fundamental vertical accuracy, achieved in such terrain, serves as a benchmark for assessing LiDAR dataset vertical accuracy. The distribution of checkpoints is contingent on land cover type and terrain, with guidelines provided by standards like the ASPRS 2014 Standard.
Relative Accuracy
Relative accuracy focuses on the internal quality of LiDAR elevation data without relying on surveyed ground control points. It assesses local differences between points in the point cloud and is influenced by LiDAR system calibration. This calibration is essential for ensuring stability both within the same swath (within-swath accuracy assessment) and between swaths or flight lines (swath-to-swath accuracy assessment).
Factors Affecting LiDAR Accuracy:
Sensor Calibration
LiDAR sensors must undergo meticulous calibration processes to ensure accurate measurements. Calibration involves aligning and adjusting various components of the sensor, such as the laser transmitter, receiver, and internal timekeeping systems. Any misalignment or error in calibration can lead to inaccuracies in the captured data.
Georeferencing (GPS and IMU Data)
Global Positioning System (GPS) data is crucial for determining the precise location of LiDAR measurements. Accurate georeferencing relies on high-quality GPS data to correctly place each point in three-dimensional space.
Inertial Measurement Units (IMUs) help account for the sensor's orientation and motion. Any errors or drift in IMU data can result in inaccuracies in the LiDAR point cloud.
Environmental Conditions
Adverse weather conditions, such as rain, fog, or snow, can scatter laser beams and interfere with the accurate detection of objects. Some LiDAR systems may incorporate algorithms to compensate for such environmental factors, but extreme conditions can still pose challenges.
Data Processing and Filtering
Proper data processing techniques, including filtering and noise reduction algorithms, are vital for cleaning and refining raw LiDAR data. Inaccuracies can be introduced during the processing stage if not handled appropriately.
While high resolution is valuable, it should not compromise accuracy. Striking the right balance is essential to ensure that the detailed information provided by high-resolution data is also trustworthy and aligns with the true characteristics of the surveyed area.
The ideal balance between resolution and accuracy depends on the specific requirements of the project. For applications where precise details are critical, a higher resolution might be prioritized. However, for tasks where overall accuracy is paramount, a balance that leans towards accuracy may be preferred.
What is the Range of Current LiDAR Mapping Systems?
LiDAR systems can detect objects at distances ranging from a few meters to more than 2,000 meters. The range of a LiDAR system depends on its design and purpose. Here are some types of LiDAR sensors and their ranges:
- Long-range LiDAR: Has a range beyond 100 meters
- Short-range LiDAR: Detects objects within 25 meters
- Mid-range LiDAR: Has a minimum resolution of about 5-10cm@30m.
- Linear LiDAR: Has a swath width of 1,000 meters.
- Geiger LiDAR: Can scan widths of 5,000 meters.
What is a DTM in LiDAR?
A Digital Terrain Model (DTM) in LiDAR applications meticulously represents the Earth's ground surface by meticulously excluding objects such as buildings and vegetation. This specialized model concentrates solely on the intrinsic topography, affording a nuanced understanding of the natural terrain. It proves invaluable in hydrological modeling, where precise delineation of ground features is pivotal for accurate water flow simulations, and in geological studies where unadulterated landform analysis is essential.
What is a DSM in LiDAR?
A Digital Surface Model (DSM) in the LiDAR context encapsulates the entirety of the Earth's surface, seamlessly integrating both the ground and above-ground elements. This model yields a holistic representation, offering rich visual detail encompassing features like buildings, trees, and other structures. DSMs find applicability in urban planning endeavors, facilitating the modeling of cityscapes, assessing building heights, and aiding in infrastructure development planning. Additionally, in forestry applications, DSMs prove instrumental for gauging canopy structures, tree heights, and overall vegetation density.
What is DEM in LiDAR?
The overarching term Digital Elevation Model (DEM) serves as a comprehensive framework that encompasses both DTMs and DSMs. Its adaptability allows it to be tailored to specific requirements, serving as a foundational dataset for various applications. In practice, DEMs can be derived from LiDAR point cloud data, incorporating either the meticulously refined terrain focus of a DTM or the all-encompassing detail of a DSM. This versatility makes DEMs fundamental for terrain analysis, including slope and aspect assessments, as well as their indispensable role in cartography, forming the basis for creating detailed topographic maps.
What is the Difference Between Radar and LiDAR?
Radar and LiDAR are technologies designed for object detection, each with its distinct features.
Radar employs radio waves to gauge the time taken for signals to return after hitting objects. This adaptability makes it ideal for various weather conditions, commonly finding use in aviation and weather monitoring due to its ability to penetrate obstacles and work in adverse weather.
LiDAR, on the other hand, uses laser light to measure distances and create detailed 3D maps. Known for its precision, LiDAR calculates distances by analyzing the time taken for laser pulses to return after hitting objects. It excels in accuracy and is extensively utilized in autonomous vehicles, environmental monitoring, and forestry, contributing to the creation of precise digital elevation models.
The choice between radar and LiDAR often depends on the specific demands of the application. Radar, with its adaptability and longer range, suits broader surveillance contexts, while LiDAR, with its precision and detailed mapping capabilities, excels in scenarios requiring accurate object recognition and intricate environmental modeling.
What is the Difference Between LiDAR and IR Sensors?
LiDAR employs laser light to measure distances and create detailed three-dimensional maps. It operates by emitting laser pulses toward objects and analyzing the time it takes for these pulses to return after hitting the objects. This precise distance measurement allows LiDAR to generate highly accurate 3D models of the surrounding environment. Its applications range from autonomous vehicles to forestry and environmental monitoring.
Infrared (IR) sensors, on the other hand, work by detecting infrared radiation either emitted or reflected by objects. These sensors are commonly used for proximity sensing, object detection, and even in night vision applications. Unlike LiDAR, IR sensors do not rely on laser light pulses but instead focus on capturing the infrared energy emitted by objects.
The primary distinction lies in their operational principles. LiDAR actively sends out laser pulses for distance measurement, enabling detailed mapping and object recognition. In contrast, IR sensors passively detect infrared radiation, making them versatile in various sensing applications, particularly in low-light conditions.
LiDAR's strength lies in its high precision and ability to create detailed 3D maps, making it suitable for scenarios where accurate distance measurements and mapping are critical. IR sensors, on the other hand, excel in applications where the focus is on detecting the presence of objects or capturing infrared radiation for specific sensing purposes.