LiDAR vs. Radar: What’s the Difference?
LiDAR and Radar are two influential remote sensing technologies, each with a distinct approach to detecting and measuring distances.
LiDAR uses laser beams to precisely gauge the time taken for light to bounce back, providing superior accuracy and intricate 3D mapping.
In contrast, Radar employs radio waves, excelling in versatility and adaptability across diverse conditions.
Both technologies have significantly impacted fields like transportation, aviation, environmental monitoring, and disaster management.
In this article, we'll dive into the core principles, advantages, drawbacks, and practical applications of LiDAR and Radar, offering insights for experts, scholars, and enthusiasts seeking to harness their potential.
If you're in a hurry, check out the table below for a quick look at the pros and cons of LiDAR and radar:
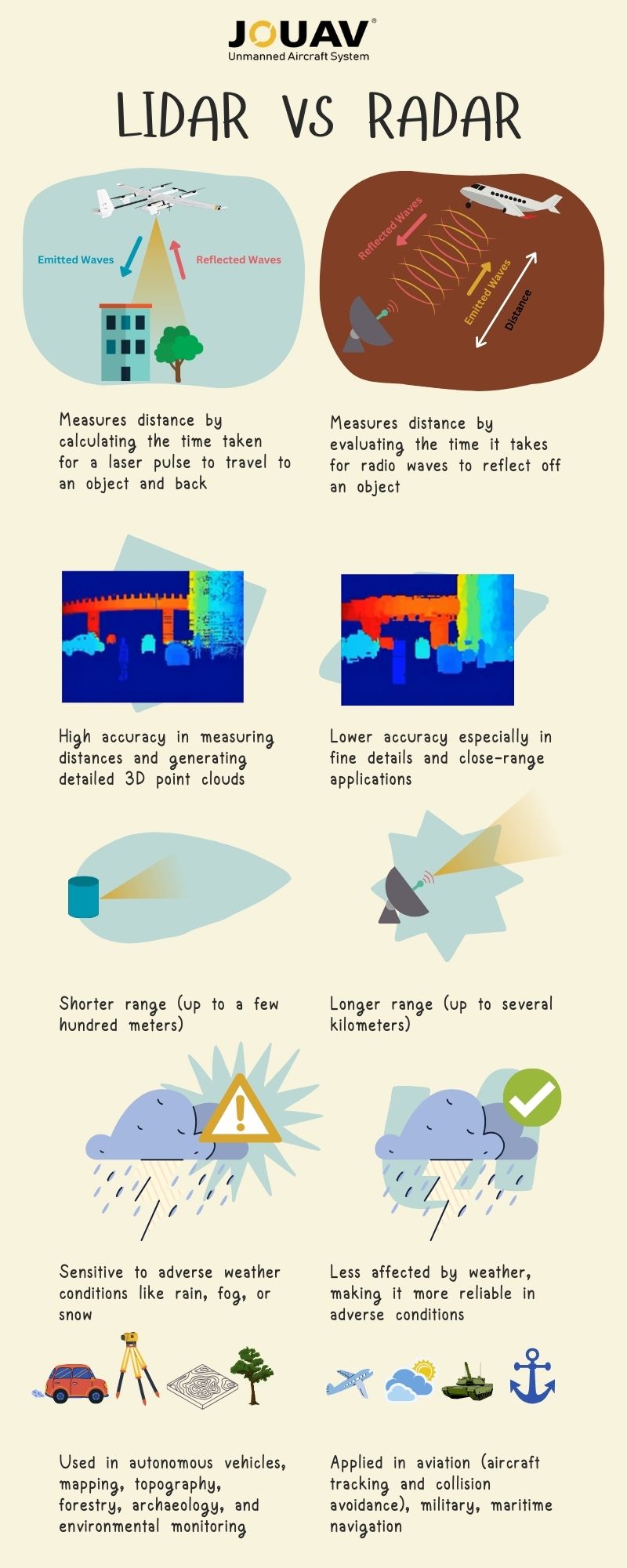
What Does LiDAR Stand for?
LiDAR, short for "Light Detection and Ranging," is a remote sensing technology that relies on laser light to measure distances accurately. This process enables the creation of highly detailed 3D maps of the environment. LiDAR systems operate by emitting rapid laser pulses and meticulously measuring the time it takes for the reflected light to return.
This technology has evolved significantly since its inception in the early 1960s, driven by advancements in lasers, computing, and data processing capabilities.
How Does LiDAR Work?
A typical LiDAR system is composed of three essential components:
- Laser Source: LiDAR systems depend on lasers to emit rapid bursts of light, typically in the near-infrared spectrum. These laser pulses traverse the atmosphere until they encounter objects like terrain features, trees, or structures.
- Scanner: To ensure thorough data collection, a scanner, often a rotating mirror or micro-electromechanical system (MEMS), directs the laser pulses in various directions.
- Detector: The detector, an incredibly sensitive instrument, gauges the time it takes for the laser pulses to travel to objects and return to the LiDAR system. This "time-of-flight" principle, combined with the constant speed of light, allows for precise distance calculations.
When a laser pulse hits an object, such as a tree or a structure, the light bounces back to the LiDAR system. The scanner, often a revolving mirror or MEMS, collects and directs the returning light to the detector. The detector is a susceptible instrument that detects the time taken for light to travel back and forth between the LiDAR system and the object. This time-of-flight principle, combined with the constant speed of light, allows LiDAR to calculate the distance to an object accurately.
How does LiDAR work? Source from Yellowscan
LiDAR devices release thousands or even millions of pulses per second to create a thorough map of the environment. This generates a massive amount of data, which is then utilized to generate a very accurate and detailed 3D map of the surroundings. The map's resolution is determined by the number of pulses emitted, the frequency of the laser, and the detector's sensitivity.
Types of LiDAR Sensors
LiDAR technology encompasses various sensor types, each tailored to specific applications, making it a versatile tool for capturing precise 3D data. Below, we explore the diverse types of LiDAR sensors and their respective applications:
Airborne LiDAR
Airborne LiDAR systems are installed on fixed-wing drones and helicopters, and they play a pivotal role in remote sensing. They emit infrared laser pulses toward the ground, capturing the reflections as the aircraft moves. There are two primary categories of airborne LiDAR sensors:
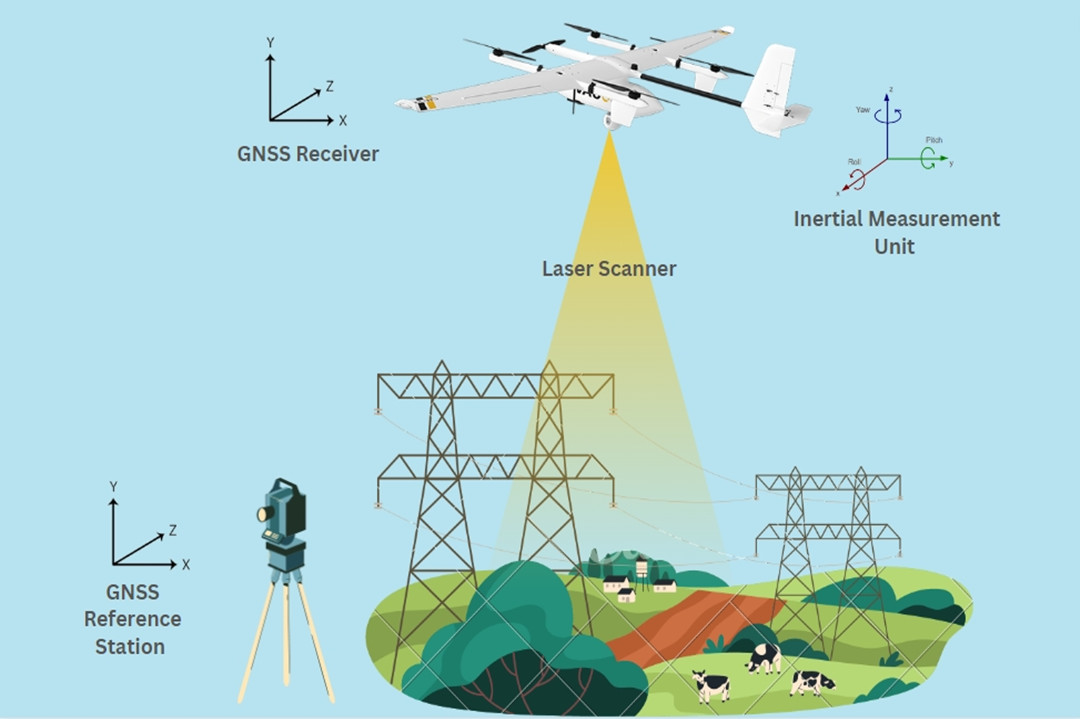
Topographic LiDAR
Topographic LiDAR is employed to derive surface models for many applications, including forestry, hydrology, geomorphology, urban planning, landscape ecology, coastal engineering, survey assessments, and volumetric calculations. It aids in creating precise representations of terrestrial landscapes and is invaluable for decision-making in various industries.
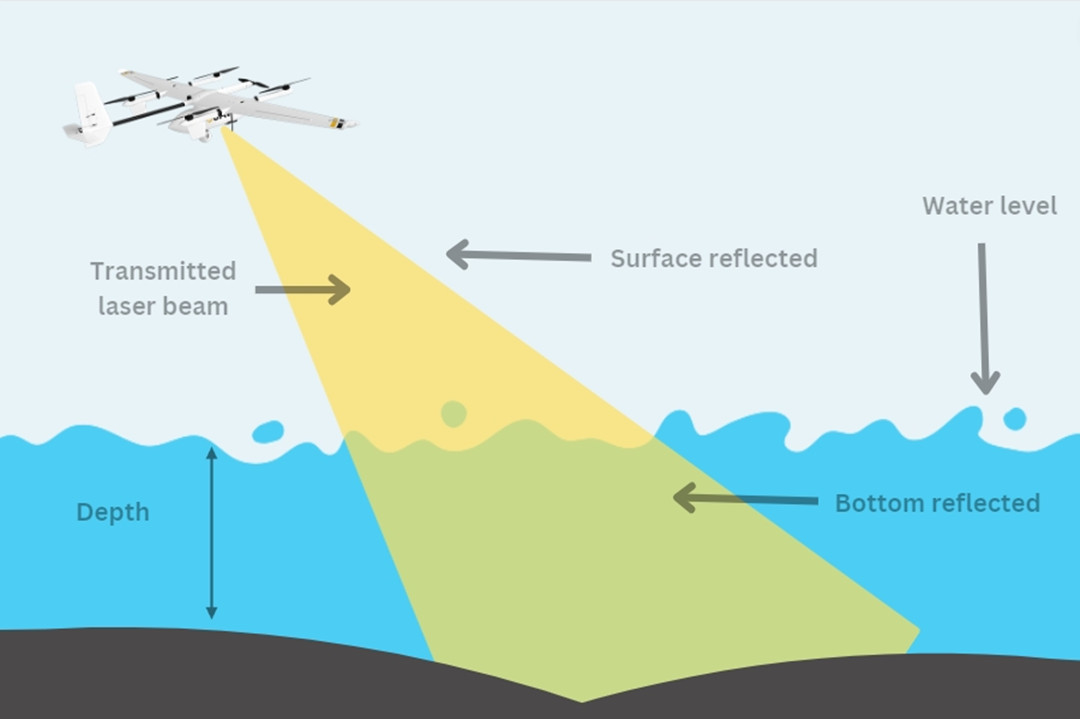
Bathymetric LiDAR
Bathymetric LiDAR is designed for water-penetrating acquisitions, collecting elevation and water depth data simultaneously. This sensor is particularly crucial for surveys of the land-water interface, especially near coastlines, harbors, shores, and banks. It facilitates the detection of objects on the ocean floor, serving marine and coastal applications effectively.
Terrestrial LiDAR
Terrestrial LiDAR can be further categorized into two main types, mobile and static, depending on their mobility and deployment methods. These sensors utilize eye-safe lasers to generate dense and highly accurate point clouds, enabling a myriad of applications:
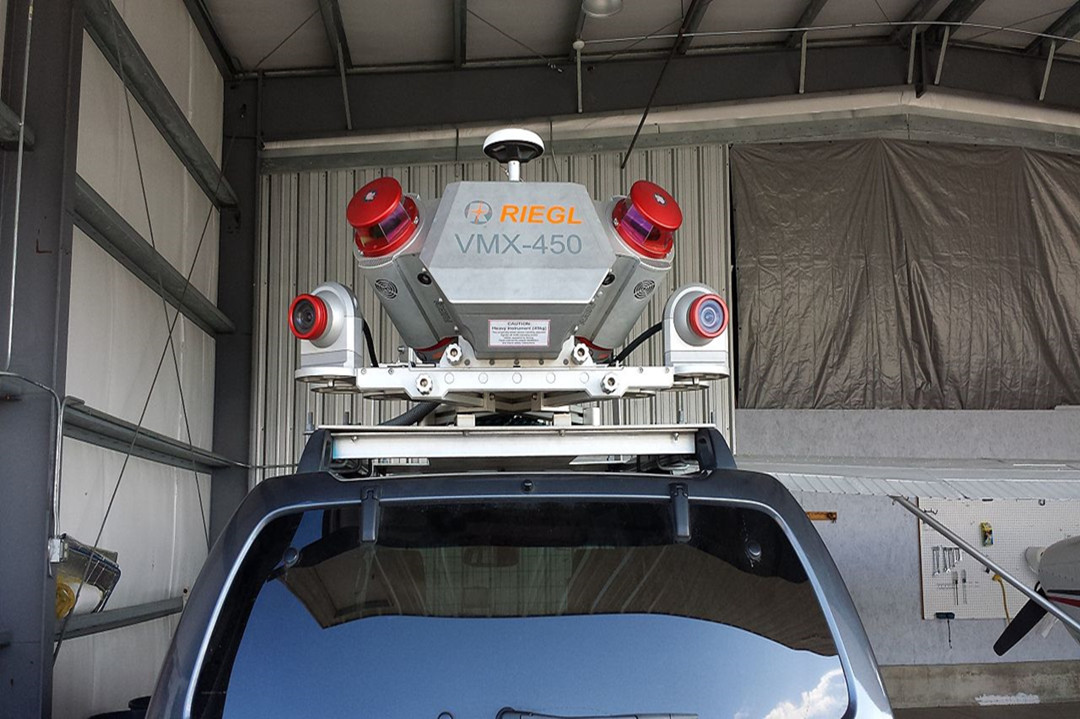
Mobile LiDAR
Mobile LiDAR systems are mounted on moving platforms, including vehicles, trains, and boats. With LiDAR sensors, cameras, GPS (Global Positioning System), and INS (Inertial Navigation System), mobile LiDAR is extensively used for infrastructure analysis, overhead wire detection, light pole identification, and sign assessment along roadways and rail lines. It finds wide-ranging applications in urban planning, transportation engineering, and infrastructure management.
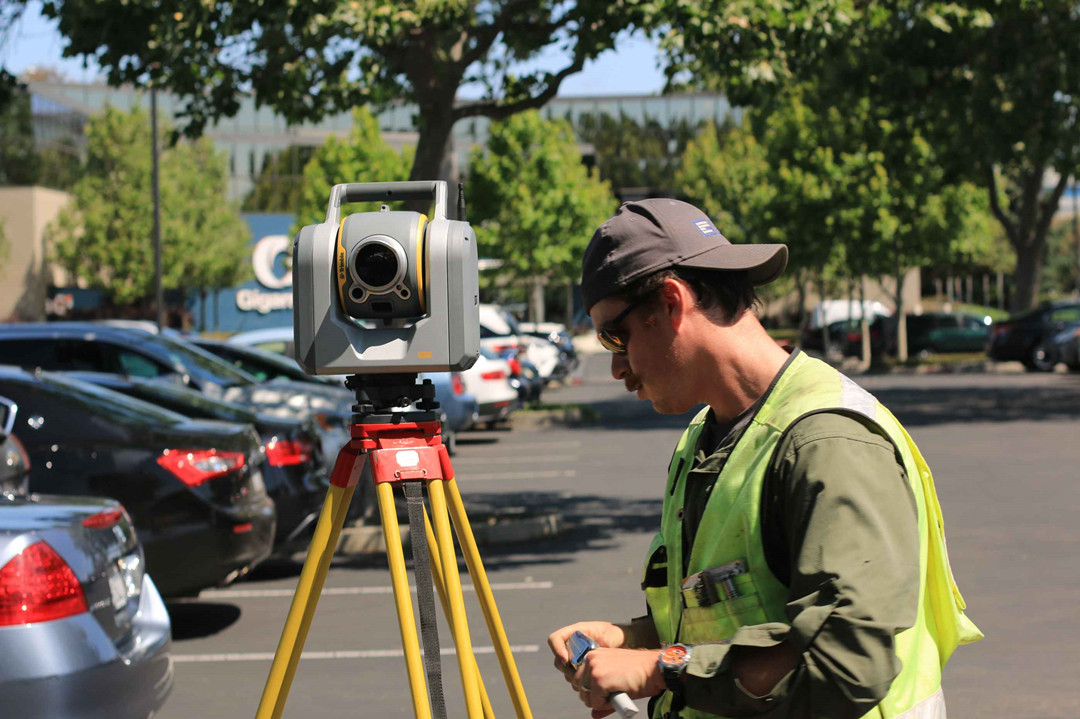
Static LiDAR
Static LiDAR sensors are deployed from a fixed location, typically mounted on a tripod or stationary device. These portable laser-based ranging and imaging systems are employed for an extensive array of applications. Engineers use static LiDAR to monitor facilities, conduct highway and rail surveys, and create detailed 3D models of urban exteriors and interiors. It is also indispensable for archaeological investigations, surveying, mining operations, and architectural planning.
LiDAR's capacity to provide high-resolution, three-dimensional data has made it a vital tool in numerous fields, including topographic mapping, forestry management, urban planning, autonomous vehicle navigation, and more. Its precision and ability to create comprehensive 3D maps have revolutionized the way we collect and interpret spatial information.
What is Radar?
Radar, which stands for "Radio Detection and Ranging," is a formidable sensing technology that relies on radio waves to detect objects and measure their distances, velocities, and various characteristics.
The essence of Radar lies in its ability to emit radio waves into the atmosphere, which then bounce back when they encounter an object. By analyzing the time taken for these radio waves to return and factoring in the frequency shift caused by the Doppler effect, Radar systems can accurately determine an object's distance, speed, and direction.
Radar has a rich history, dating back to its early development in the 20th century. Since then, this technology has undergone continuous refinement and improvement, earning its place in various industries, including aviation, meteorology, and military surveillance.
How Does Radar Work?
A typical Radar system consists of several key components, including:
- Antenna: The antenna is the part of the Radar that transmits and receives radio waves. It emits a radio signal and then captures the reflected waves.
- Transmitter: The transmitter generates the radio frequency signal, which is sent out by the antenna. The emitted radio waves travel through the air until they encounter an object.
- Receiver: The receiver is responsible for capturing the reflected radio waves after they bounce off an object. By analyzing the time it takes for the waves to return and their frequency shift (Doppler effect), Radar can calculate the distance, speed, and other characteristics of the detected object.
- Signal Processor: Radar data is processed to create a visual representation or provide information about the detected objects. Signal processing is crucial for extracting valuable information from the returned radio waves.
The electromagnetic energy used in radar travels through the air at a constant speed, approximately the speed of light (300,000 kilometers per second or 186,000 miles per second). By measuring the time between transmitting and receiving energy pulses, radar systems precisely determine the distance between the reflecting object - whether airplanes, ships, or cars—and the radar site.
Radar performance can be influenced by factors such as operating frequency, atmospheric conditions, and interference from electronic devices and obstacles. The radar's choice of frequency affects its ability to penetrate materials and its susceptibility to signal interference. Atmospheric conditions, like precipitation, can reduce radar accuracy and range.
Types of Radar Systems
Radar technology has evolved into a spectrum of varieties, each tailored for specific configurations, scanning patterns, and applications. While basic pulsed and monostatic Radar systems are the building blocks, more sophisticated options abound, including:
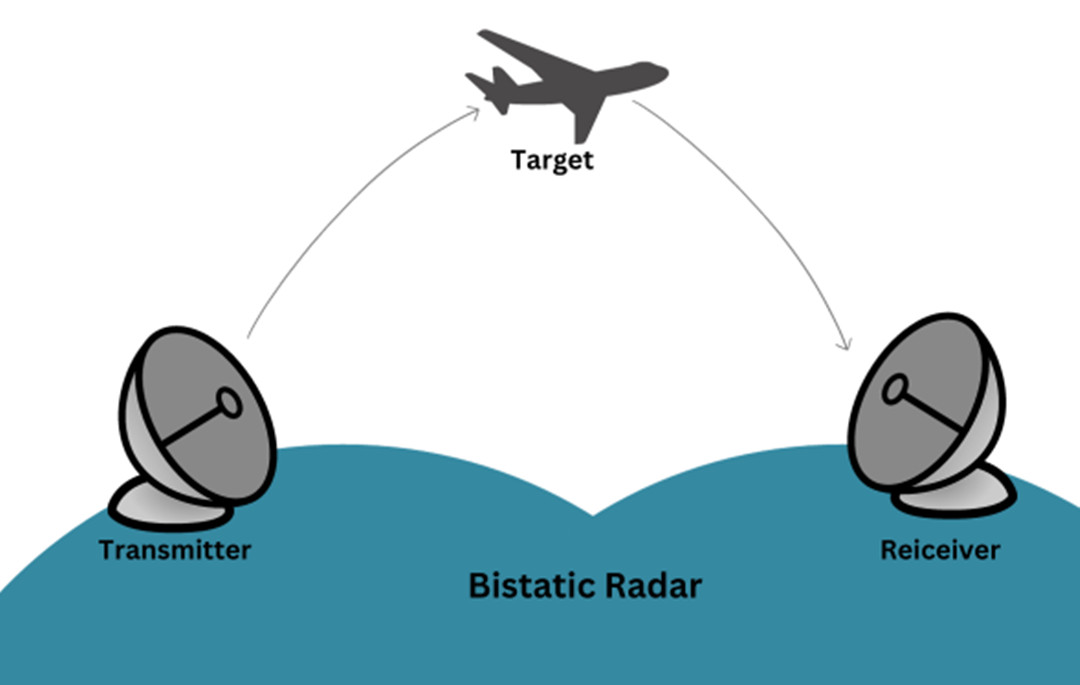
Bistatic Radar
Bistatic Radar systems consist of a static transmitter and a static receiver, positioned at a significant distance from each other, roughly matching the expected target distance. These systems excel when the target reflects minimal energy toward the receiver, such as in modern stealth technology-equipped fighter jets or in meteorological applications.
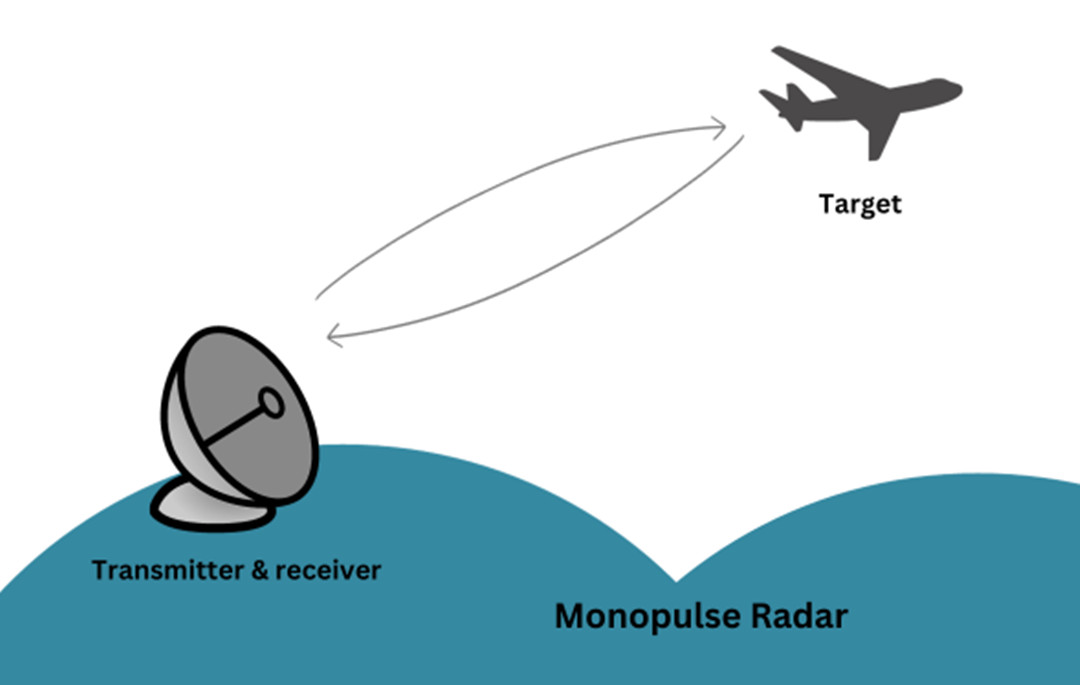
Monopulse Radar
Monopulse Radar employs single, narrow pulses of electromagnetic energy to accurately gauge an object's distance and direction. It sends out energy signals that split into two beams extending to each side of the intended target. Analyzing the difference between the two reflected signals determines the precise direction of the object. Monopulse Radar systems are advanced and widely used in high-accuracy military applications.
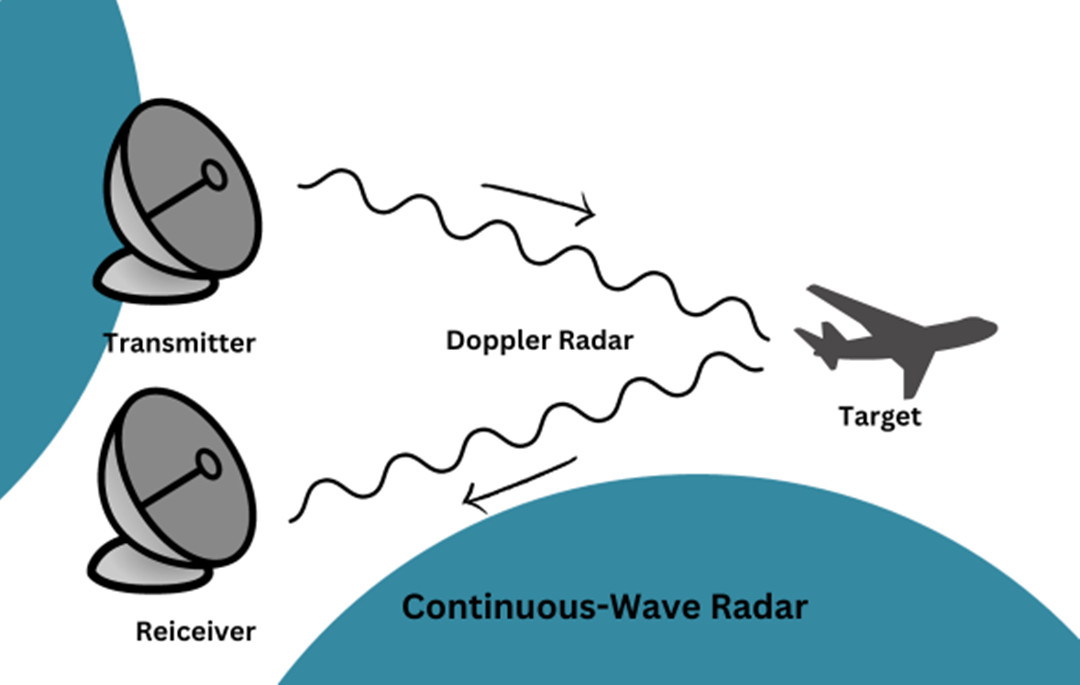
Continuous-Wave Radar (CW)
Continuous-wave radar constantly emits electromagnetic energy, unlike traditional pulsed systems. These systems continuously receive reflected energy and are exceptionally effective at detecting motion, allowing for the tracking of moving objects while ignoring stationary ones, like the ground beneath low-flying aircraft. Their simplicity makes CW Radar systems cost-effective, reliable, and compact. Some CW Radar systems can reliably detect moving objects over 100 km away.
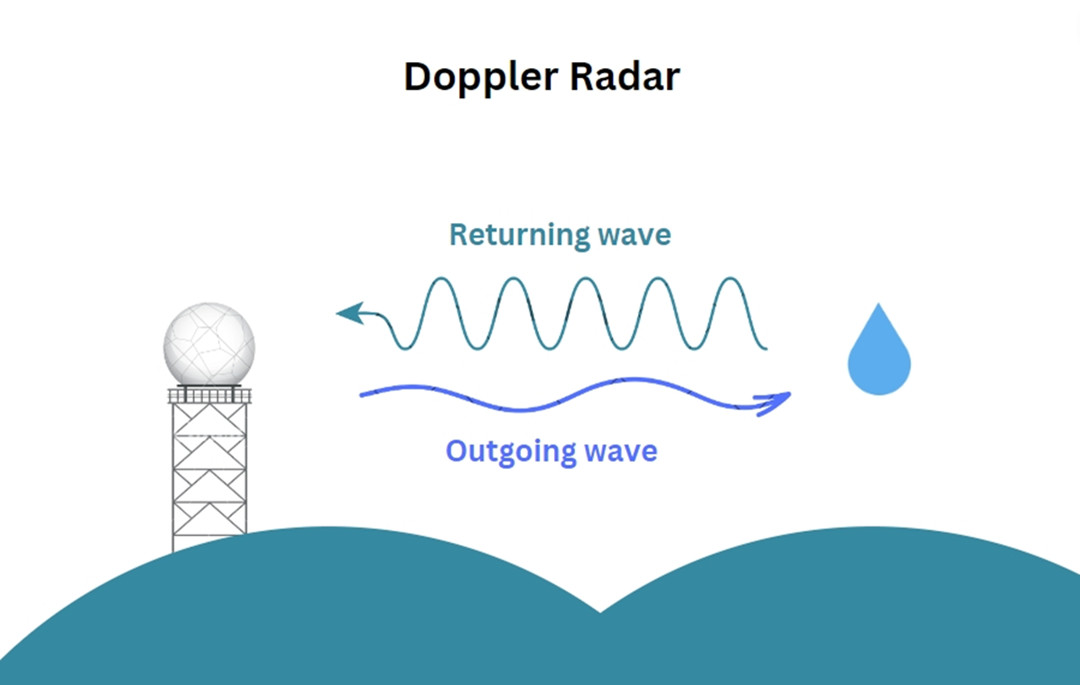
Doppler Radar
Doppler Radar is a variation of CW Radar that leverages the Doppler effect to determine the velocity of detected moving objects. The Doppler effect describes the change in frequency of electromagnetic waves as they bounce off objects. When the Radar system receives echoes with a different frequency than the transmitted waves, it calculates the object's velocity. Doppler Radar finds applications in aviation, meteorology, sports analysis (e.g., Major League Baseball's Statcast), healthcare, and missile defense systems.
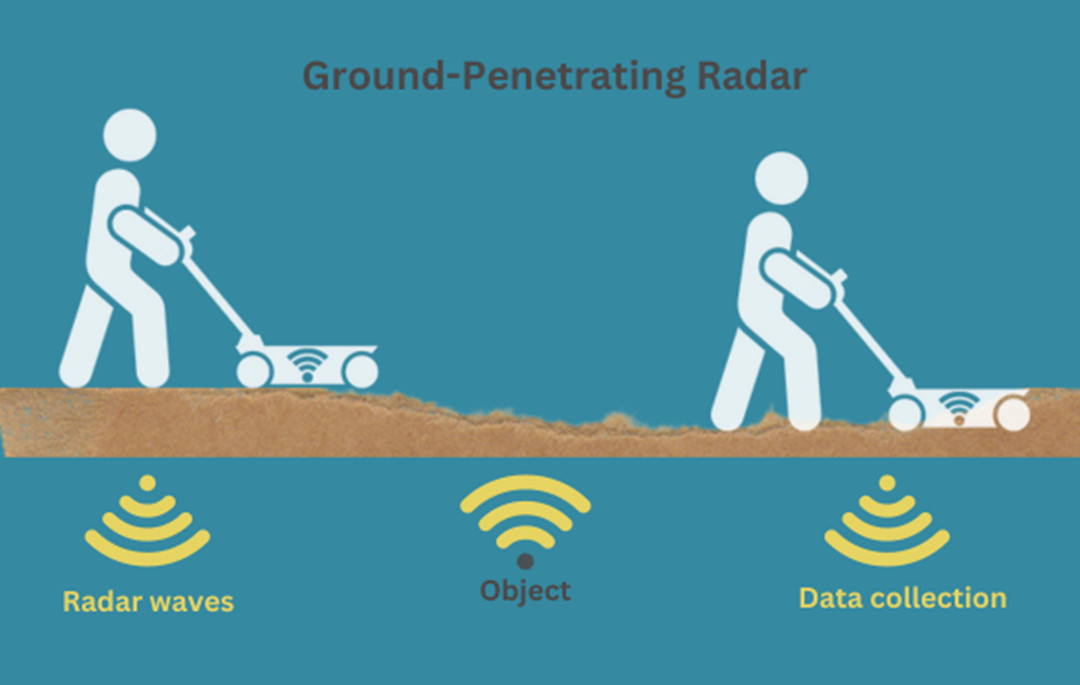
Ground-Penetrating Radar (GPR)
Ground-penetrating radar scans the ground surface to identify materials and objects buried underneath. It is commonly used to locate and identify utility systems beneath the ground and can detect various ground characteristics, such as density, composition, and water content. Depending on the ground composition and frequencies used, GPR can measure up to 15 meters below the surface.
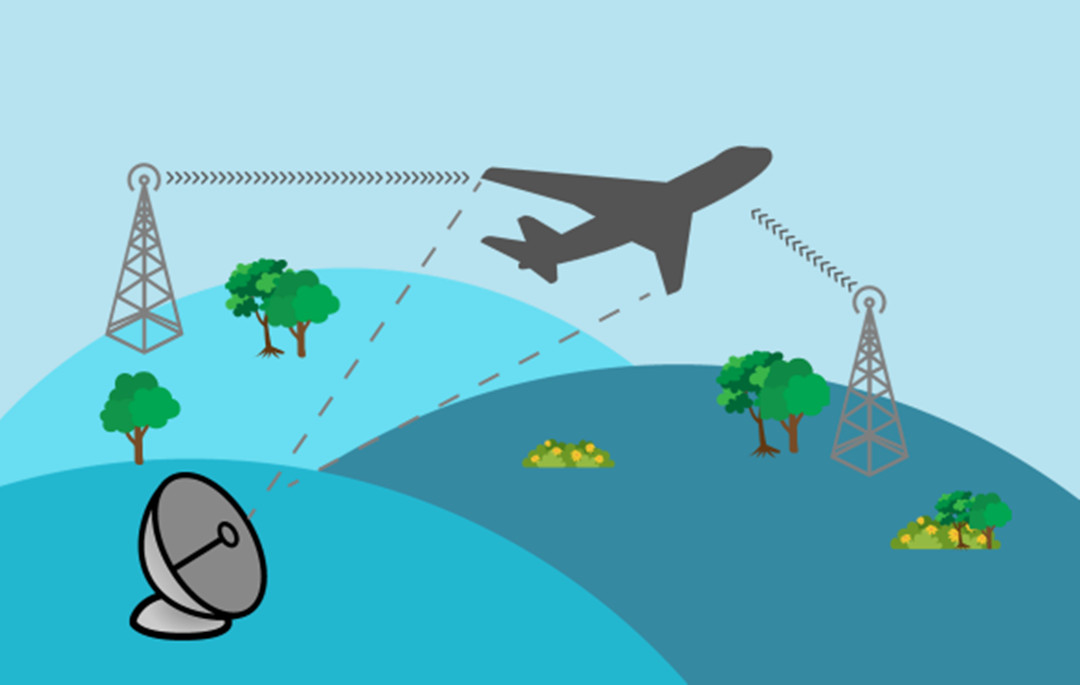
Passive Radar
Passive Radar systems use radio waves from sources like TV and FM radio to detect reflections from flying objects. Unlike traditional Radar, these systems operate covertly, making them useful for aircraft detection and meteorology. Processing signals for presence, direction, and velocity poses challenges, but passive Radar has become a viable option in the 21st century.
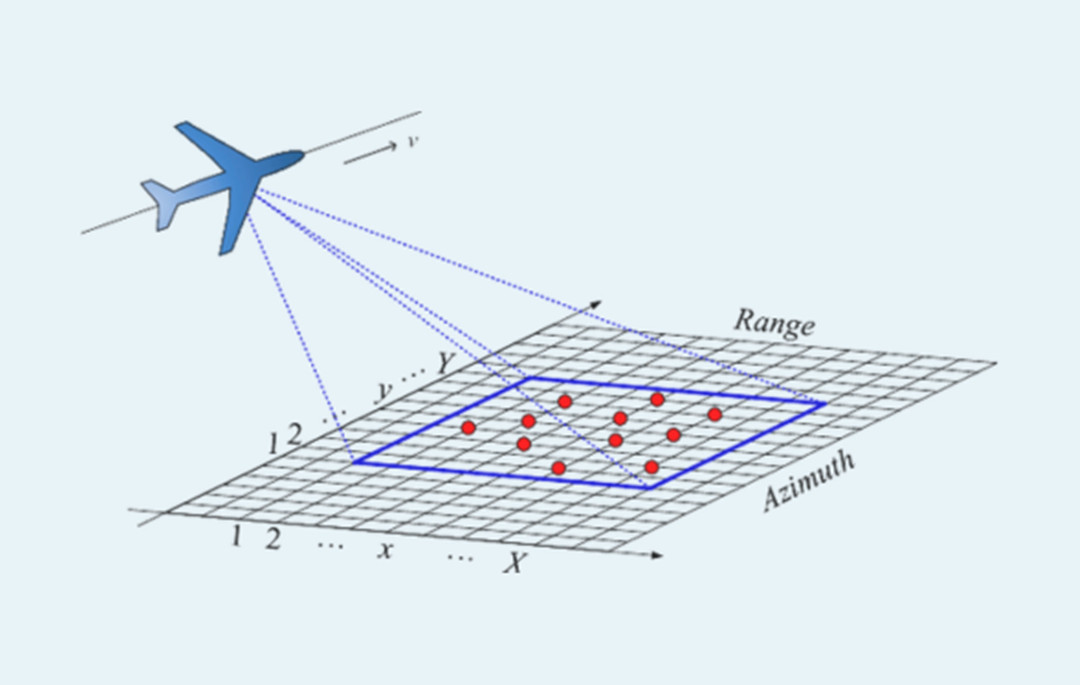
Synthetic-Aperture Radar (SAR)
Synthetic-aperture radar (SAR) systems specialize in broad-area terrain imaging, capturing high-resolution images for applications like geology, environmental monitoring, military reconnaissance, and more. These systems provide quality imaging irrespective of the time of day or atmospheric conditions. While SAR systems are costlier compared to other Radar types, recent years have seen explorations into their use for smaller-scale applications.
Radar technology is a versatile and robust tool, capable of serving diverse industries and applications. Whether it's monitoring weather patterns, guiding aircraft safely, or enhancing military surveillance, Radar continues to be a vital asset, contributing to scientific research and practical applications.
What is the Difference Between LiDAR and Radar?
When comparing LiDAR (Light Detection and Ranging) and Radar (Radio Detection and Ranging) technologies, it's essential to delve into their fundamental principles, operational methodologies, and performance characteristics comprehensively.
Accuracy and Resolution
LiDAR is known for its high granularity and exceptional accuracy. It emits laser pulses and meticulously measures the time it takes for the light to return. This results in highly accurate 3D point clouds, making LiDAR ideal for applications demanding precise spatial information. Its accuracy can reach centimeter-level precision, providing intricate details about the environment.
Radar, while offering good accuracy, typically has lower resolution compared to LiDAR. Radar relies on radio waves, which have much larger wavelengths, leading to less detailed data. While Radar can provide accurate distance and velocity measurements, it may struggle in scenarios requiring fine-scale information. Its accuracy is generally in the meter range, which is less precise compared to LiDAR.
Range and Long-Distance Detection
LiDAR excels in short to medium-range applications. Its effective range is limited by the speed of light, typically up to a few kilometers. While some LiDAR systems have extended ranges, they are less common and may come at a higher cost. Therefore, it's well-suited for applications like autonomous vehicles, environmental monitoring, and infrastructure assessment.
Radar, on the other hand, is renowned for its extended detection range. It can effectively detect objects at long distances, making it a preferred choice for applications such as air traffic control, weather surveillance, and military reconnaissance. Its range can extend to tens of kilometers, enabling it to cover vast areas effectively.
Speed Detection and Measurement
LiDAR primarily measures distance and is not typically used for speed detection. The frequency of LiDAR systems falls within the optical spectrum, usually in the infrared range. Thus, it lacks the capacity for direct speed measurement.
Radar excels at speed detection and measurement. Doppler Radar, in particular, utilizes the Doppler effect to accurately determine the velocity of moving objects.
Radar systems operate in the radio frequency spectrum, which allows for reliable speed calculations. This capability is vital for applications like traffic enforcement, aviation, and military target tracking.
Reliability and Environmental Adaptability
LiDAR systems, which rely on light waves, are more susceptible to interference from atmospheric conditions than Radar systems. The presence of fog, rain, or snow can scatter, absorb, or reflect the emitted laser light, leading to reduced measurement accuracy and overall system performance. For instance, the attenuation of laser light in fog can be as high as 200 dB/km, substantially limiting the effective range and resolution of a LiDAR system under such conditions.
In contrast, Radar systems, which use radio waves, are generally more robust against adverse weather conditions. Radio waves have longer wavelengths than light, allowing them to penetrate through various materials, including fog, rain, and snow, with less attenuation. As a result, Radar systems can maintain their performance under challenging weather conditions, providing more reliable and consistent data. This characteristic is particularly advantageous for applications that require continuous operation, such as air traffic control and meteorological monitoring.
Cost and Complexibility
LiDAR systems are generally more expensive and complex than Radar systems. The higher cost of LiDAR systems is attributed to several factors, including the need for high-precision lasers, sensitive detectors, and advanced data processing capabilities. Additionally, the mechanical components required for the scanning process, such as rotating mirrors or optomechanical systems, contribute to the overall complexity and cost of the system.
Moreover, the high-resolution data generated by LiDAR systems demands more processing power and storage capacity, leading to increased costs for data management and analysis. Furthermore, the sensitivity of LiDAR systems to environmental conditions may necessitate more frequent calibration and maintenance to ensure optimal performance.
Radar systems, on the other hand, are generally less expensive and less complex than LiDAR systems. The primary components of a radar system, such as the transmitter, receiver, and antenna, are typically more straightforward and cost-effective to produce compared to the high-precision lasers and detectors required for LiDAR.
Radar systems do not typically require complex mechanical components for scanning, as electronic beamforming can be used to steer the radar beam without the need for moving parts. Operational and maintenance costs associated with Radar systems are also generally lower than those of LiDAR systems. Radar systems are more robust in adverse weather conditions, reducing the need for frequent calibration and maintenance.
LiDAR and Radar are both versatile technologies with numerous real-world applications and use cases. Here's a brief overview:
LiDAR has a wide range of applications across various industries due to its ability to create highly accurate 3D maps and models. Here are some key LiDAR applications:
Topographic Mapping
LiDAR has become a cornerstone in topographic mapping. Airborne LiDAR systems, mounted on aircraft, rapidly capture high-resolution elevation data. This enables the creation of precise and detailed digital elevation models (DEMs) covering vast areas.
Topographic mapping is essential for various sectors, including forestry, hydrology, urban planning, geomorphology, and coastal engineering. LiDAR-derived DEMs are invaluable for flood risk assessment, infrastructure development, and accurate volumetric calculations.
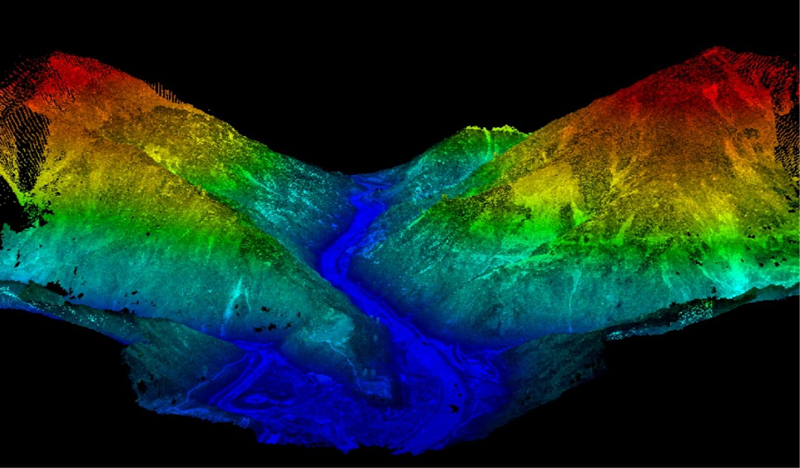
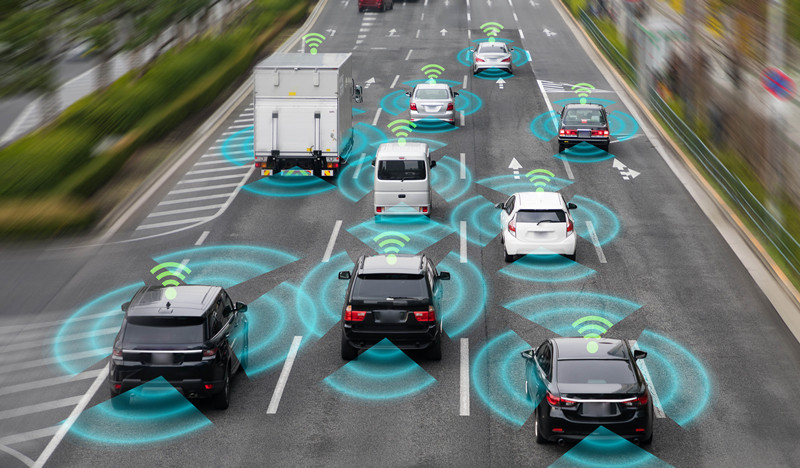
Autonomous Vehicles
LiDAR sensors, typically mounted on a vehicle's roof or sides, emit laser pulses that bounce off objects, and the time it takes for the light to return is used to calculate distances. This, coupled with data from other sensors like GPS and cameras, creates a real-time 3D map of the surroundings.
LiDAR plays a pivotal role in autonomous vehicles by offering accurate and detailed information about the vehicle's environment, allowing it to detect and avoid obstacles, even in low-light or adverse weather conditions. This technology excels at detecting pedestrians, cyclists, and other objects that may be challenging for other sensors.
Archaeology and Cultural Heritage
In the field of archaeology and cultural heritage preservation, LiDAR is invaluable. Its laser pulses create high-resolution, non-invasive 3D maps of terrain and objects on the ground.
LiDAR can reveal hidden structures, ancient settlements, and landscape features that are hard to detect using traditional surveying methods. This technology allows for the study and documentation of archaeological sites without causing damage to delicate artifacts or structures.
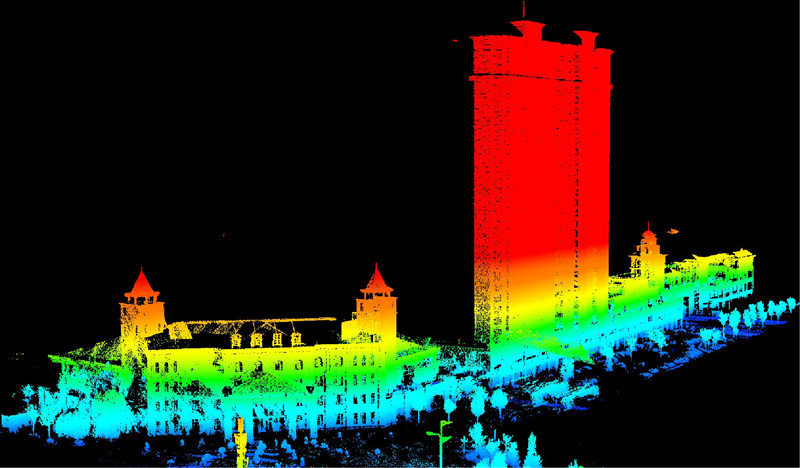
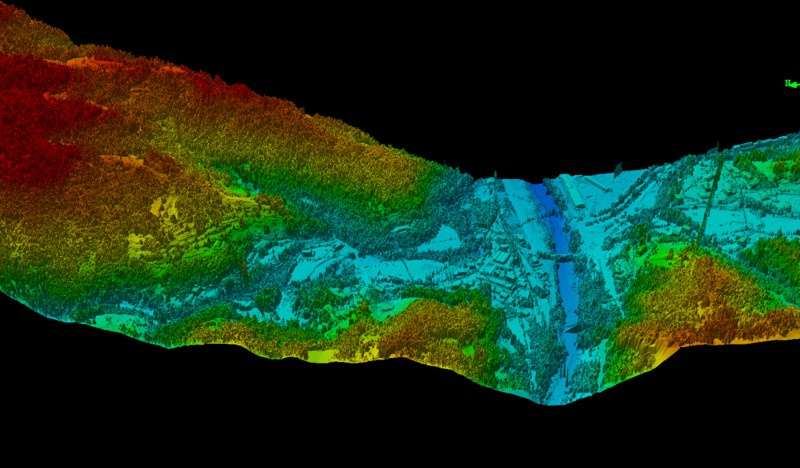
Forestry and Vegetation Analysis
LiDAR is widely used in forestry to assess the health, composition, and biomass of forests. It penetrates the tree canopy to provide detailed information on forest structure, including tree height, crown diameter, and leaf area index.
This data is crucial for monitoring forest health, managing resources, and supporting conservation efforts. LiDAR helps identify areas at risk of erosion, landslides, or other natural hazards and is also used to locate suitable sites for forest roads and infrastructure with minimal environmental disruption.
Radar technology has a broad spectrum of applications in various fields due to its ability to detect, track, and measure objects using radio waves. Here are some key radar applications:
Aviation Safety
Radar is fundamental for aviation safety. Ground-based radar systems track aircraft on the ground and in the air, ensuring safe separation. Airborne radar systems help pilots navigate and avoid weather hazards.
Advanced radar technologies, such as Automatic Dependent Surveillance-Broadcast (ADS-B), allow aircraft to transmit their position and other data to ground-based receivers for enhanced situational awareness.
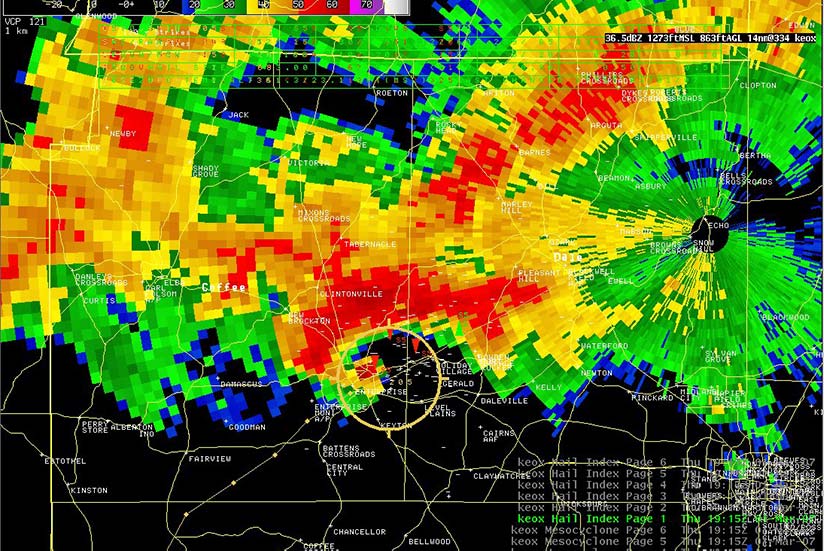
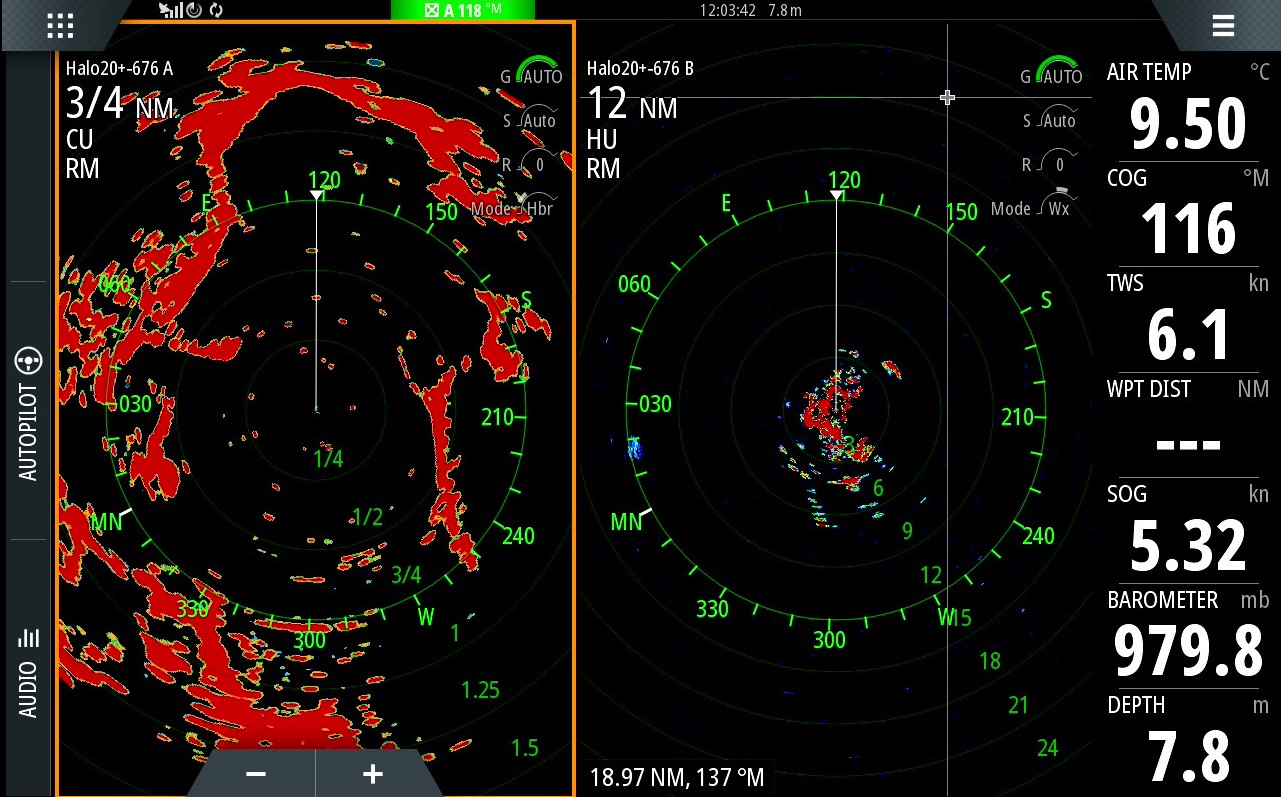
Maritime Navigation
Ships rely on radar for safe navigation, detecting other vessels, obstacles, and navigational aids like buoys and lighthouses.
Radar is crucial, especially in congested shipping lanes and low-visibility conditions, ensuring collision avoidance and safe maritime passage.
Weather Forecasting and Meteorology
Radar systems are the backbone of weather forecasting and meteorology. They detect and track weather phenomena like precipitation, storms, and tornadoes.
Radar's ability to provide real-time data on the location, intensity, and movement of weather events is essential for issuing accurate forecasts and warnings, and safeguarding lives and property.
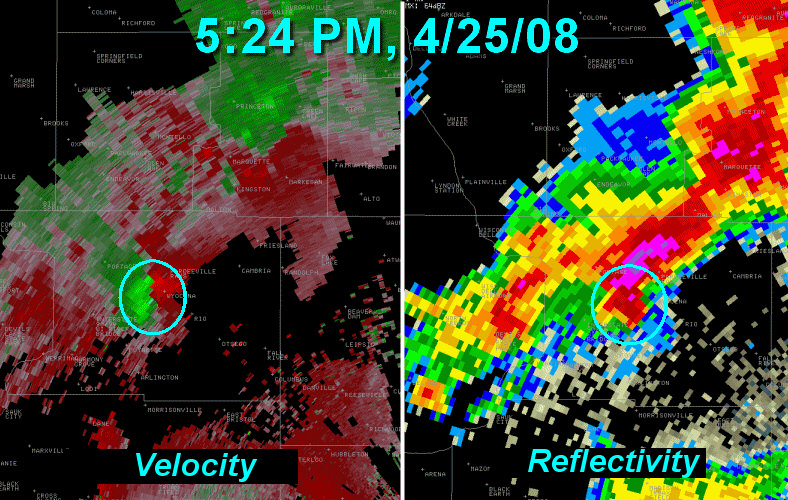
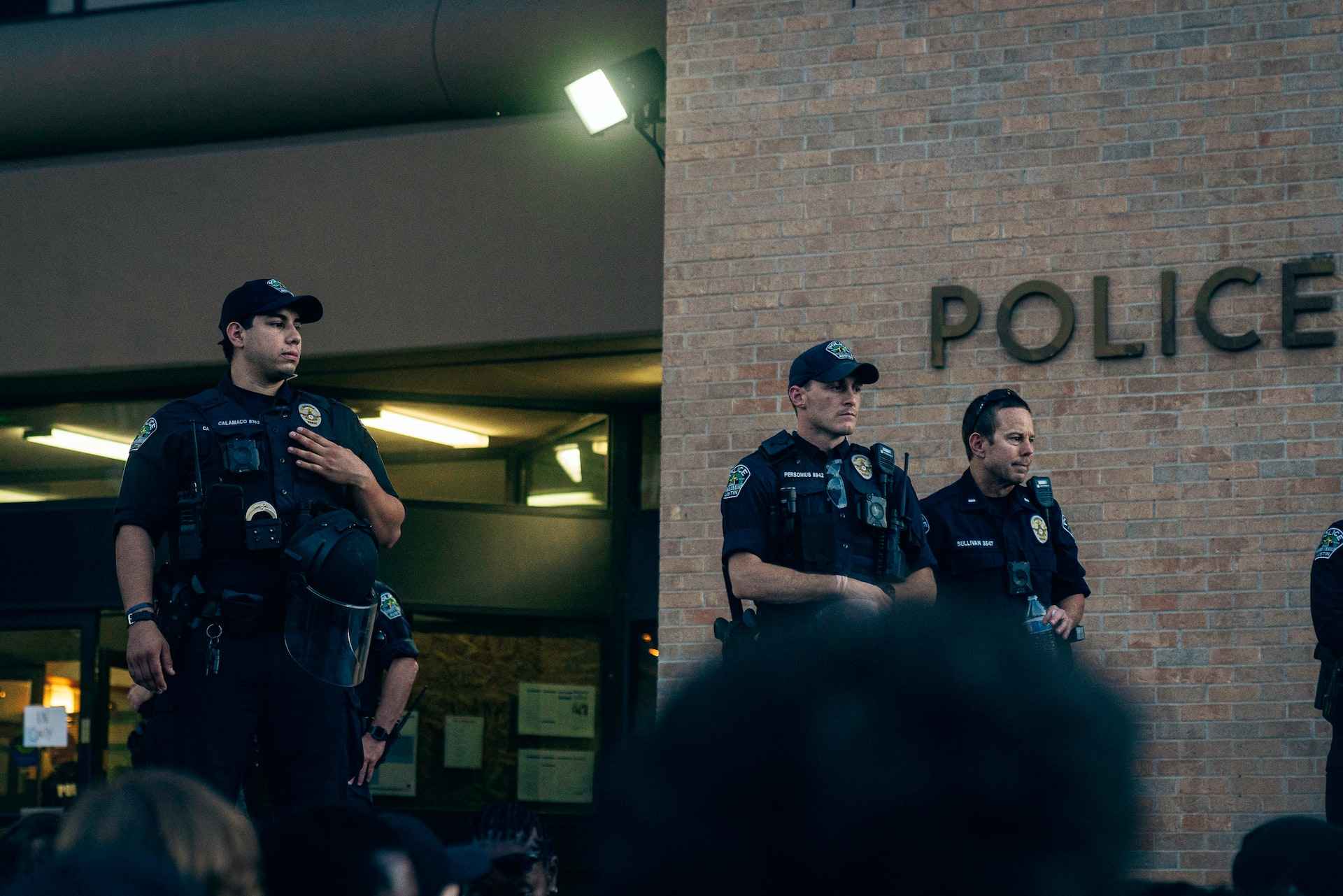
Military and Defense
Radar has been a cornerstone in military applications, aiding surveillance, target detection and tracking, missile guidance, and air defense.
Advanced radar types, including Synthetic Aperture Radar (SAR), provide high-resolution imaging and target recognition, bolstering military intelligence and situational awareness.
Automotive Safety Systems
Radar technology is integrated into automotive safety systems to improve vehicle safety and support advanced driver assistance systems (ADAS).
It enables features such as adaptive cruise control, collision avoidance, and lane departure warning systems. Radar systems detect nearby vehicles, pedestrians, and obstacles, enhancing driver awareness and reducing the risk of accidents.
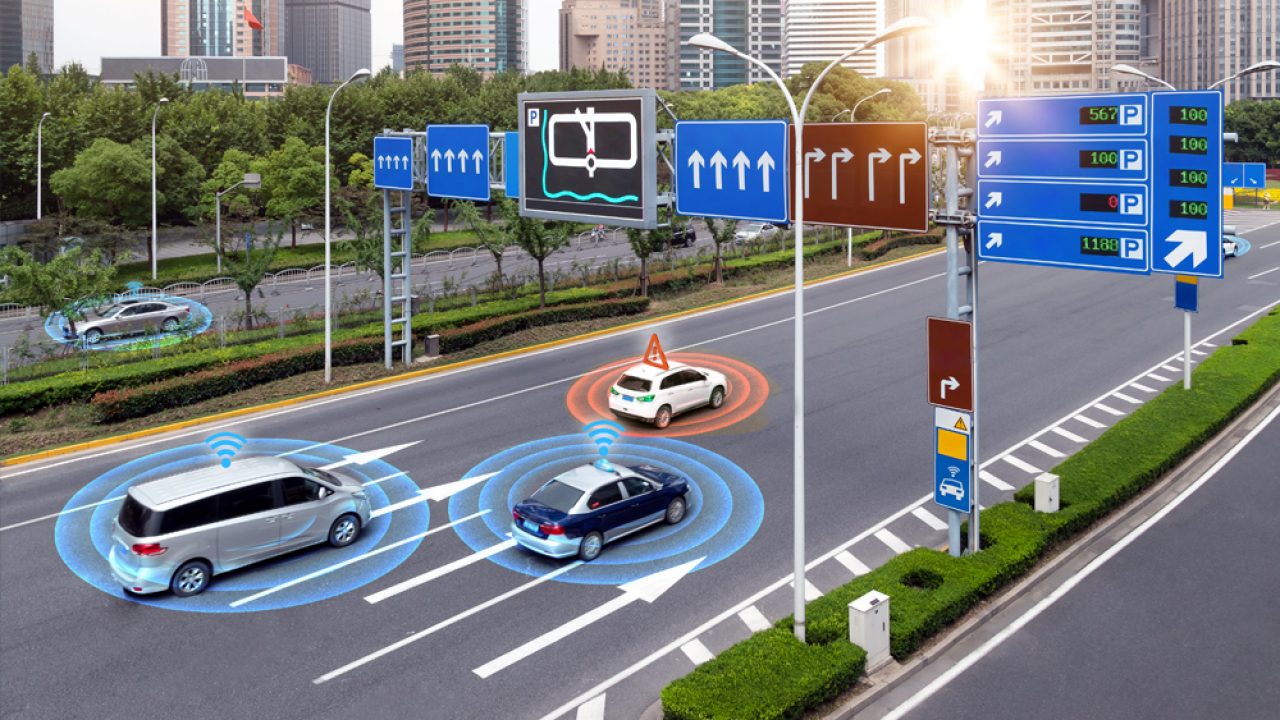
What's the Difference Between Synthetic Aperture Radar (SAR) vs LiDAR?
Synthetic Aperture Radar (SAR) and LiDAR are two distinct remote sensing technologies that serve different purposes and work based on different principles.
- Principle of Operation: SAR uses microwave radar signals to create 2D or 3D images by bouncing signals off the Earth's surface. LiDAR employs laser pulses to measure distances and create highly detailed 3D maps.
- Wavelength: SAR uses microwaves, while LiDAR uses laser light with short wavelengths.
- Environmental Interaction: SAR can penetrate clouds and vegetation, sensitive to surface moisture and roughness. LiDAR primarily operates in clear conditions and excels at capturing fine surface details.
- Resolution: SAR offers high-resolution images, dependent on radar frequency and antenna size. LiDAR is known for exceptionally high spatial resolution, capturing fine details.
- Coverage: SAR is suitable for large-scale area mapping and monitoring changes in wide regions. LiDAR is often used for localized and detailed mapping.
- Weather and Day/Night Operation: SAR works in all weather conditions and day or night. LiDAR can be affected by adverse weather and operates in daylight.
How Does Sonar Technology Work, and How Does it Differ from LiDAR and Radar?
Sonar, or "Sound Navigation and Ranging," works by emitting sound waves into water. When these waves encounter objects, they bounce back to the sonar system.
Measuring the time it takes for the waves to return allows the system to calculate distances and create underwater maps.
Differences from LiDAR and Radar:
- Medium: Sonar works underwater, LiDAR in the air or on land, and Radar in the atmosphere.
- Wavelength: Sonar uses sound waves, LiDAR employs laser light with short wavelengths, and Radar uses longer radio waves.
- Applications: Sonar is for underwater tasks. LiDAR creates 3D land maps, and Radar is versatile for atmospheric applications.
- Propagation Speed: Sound waves in water are slower than electromagnetic waves in LiDAR and Radar.
- Environmental Factors: Sonar excels in poor underwater visibility, while LiDAR and Radar can be affected by atmospheric conditions like fog or rain.
What is the Difference Between Radar and LiDAR and Ultrasonic Sensor?
Radar and LiDAR are active sensing technologies that employ radio waves and laser light, respectively, for object detection. Ultrasonic sensors use high-frequency sound waves (ultrasonic waves) to detect objects. These sensors emit sound waves and measure the time it takes for these waves to bounce off objects and return to the sensor.
Ultrasonic sensors are typically employed for close-range applications, such as obstacle detection and proximity sensing in robotics, parking assistance systems, and certain industrial automation tasks. While they are cost-effective and reliable for short distances, they may not provide the same level of accuracy and long-range capabilities as Radar and LiDAR.
LiDAR vs Radar vs Camera, Which is the Right Choice for Autonomous Vehicle?
Autonomous vehicles rely on a combination of sensor technologies, with LiDAR, Radar, and Cameras being the most prominent options. Each of these sensor types has its own set of advantages and challenges.
LiDAR employs laser beams to provide precise distance measurements and creates detailed 3D maps of a 360-degree view around the vehicle. It's highly accurate but can be expensive.
Radar uses radio waves for long-range object detection, especially useful in adverse weather conditions. It's cost-effective but provides less detailed object information.
Cameras capture visual data, aiding in object recognition, traffic sign detection, and more. They're cost-effective but can be affected by poor lighting and weather conditions.
In practice, many autonomous vehicles combine these sensors to leverage their strengths and ensure reliability in various driving scenarios. The choice depends on specific needs, cost considerations, and performance requirements.
FAQ
Are There Any Similarities Between LiDAR and Radar Technologies?
Indeed, there are similarities between LiDAR and Radar technologies. They both share some fundamental principles:
- Both rely on emitting signals and measuring the reflections to gauge distances and characteristics of objects.
- They fall under the category of active sensing technologies, meaning they generate their own signals for object detection, unlike passive sensors that rely on ambient signals.
- Both are frequently used for remote sensing applications like autonomous vehicles, aviation, and environmental monitoring.
What is the Difference Between a LiDAR and a Laser Scanner?
Laser scanners and LiDAR are both laser-based technologies, but they have distinct characteristics and applications.
Laser Scanners use multiple laser beams to capture data in a 2D view, making them efficient for quick data collection over large areas. They are often used in applications like industrial inspection and surveying.
LiDAR (Light Detection and Ranging), on the other hand, uses a single, focused laser beam to create detailed 3D maps, measuring the time it takes for laser pulses to return from objects. LiDAR is ideal for applications that demand high precision and accuracy, such as topographic mapping, urban planning, forestry management, and autonomous vehicles. It excels in capturing fine details and providing a 360-degree view of the environment.
Is LiDAR the Same as Infrared?
LiDAR (Light Detection and Ranging) and Infrared are two distinct technologies with different applications and principles of operation.
LiDAR utilizes laser pulses in the form of visible or near-infrared light to measure distances. It sends out laser beams, measures the time it takes for them to bounce off objects, and uses this data to create detailed 3D maps of the environment. It's commonly used in applications like autonomous vehicles, forestry management, urban planning, and archaeology due to its ability to provide high-precision 3D mapping.
Infrared, on the other hand, refers to a range of electromagnetic radiation with longer wavelengths than visible light. Infrared sensors detect variations in temperature by measuring the heat emitted by objects within the infrared spectrum.
This technology is applied in various applications, including night vision devices, thermal imaging cameras, temperature sensors, and remote controls. Infrared sensors are primarily used for detecting heat or temperature differences and are not typically employed for distance measurement or 3D mapping as LiDAR is.
What's the Difference Between 2D and 3D LiDAR?
2D LiDAR typically emits a single beam that scans in a horizontal plane. The sensor can rotate or tilt to collect X and Y coordinates, effectively providing a 2D representation of the environment.
2D LiDAR is often used for basic obstacle detection and mapping. It's commonly found in robotics, industrial automation, and some autonomous vehicles to provide a basic understanding of the environment, such as detecting obstacles in a single plane.
In contrast, 3D LiDAR employs multiple beams that scan not only in the horizontal (X and Y) plane but also in the vertical (Z) plane. This allows it to capture data in three dimensions, providing X, Y, and Z coordinates. This richer data set is what enables 3D LiDAR to create detailed 3D representations of the environment, offering a more comprehensive view compared to 2D LiDAR.
3D LiDAR is crucial for applications that require detailed 3D mapping, such as autonomous vehicles, urban planning, forestry management, and archaeology. It offers a comprehensive understanding of the surroundings, allowing for precise object recognition and navigation.
Is LiDAR the Same as 4D Imaging Radar?
LiDAR and 4D Imaging Radar are both crucial sensors for autonomous vehicles, but they have differences in capabilities.
LiDAR uses laser beams to create dense and accurate 3D maps, meeting the high requirements for forward detection accuracy. Its detailed point clouds help identify objects' size, distance, orientation, and speed.
4D Imaging Radar incorporates time, providing object velocity and angle data. While it's an upgrade from traditional radar, it falls short of LiDAR's point cloud density and quality. It might replace traditional radar but is not yet a match for LiDAR in high-level autonomous driving applications, especially in forward detection accuracy.
Does Police Radar Work in the Rain?
Yes, police radar can operate effectively in the rain. Radar systems used for speed detection by law enforcement are designed to function in various weather conditions, including rain. The microwave radar signals they employ are less affected by rain compared to certain other sensing technologies, making them suitable for use in inclement weather.
Which Sensor Provides the Best Resolution for Radar Imaging?
The resolution of radar imaging depends on factors like the wavelength of radar signals and antenna size. In general, shorter wavelengths and larger antennas result in higher resolution. Synthetic Aperture Radar (SAR) systems, for instance, can provide very high-resolution radar images by synthesizing a large antenna aperture over a relatively long path.
Do Cars Use Radar or LiDAR?
Different car manufacturers employ various sensor combinations for advanced driver assistance systems (ADAS) and autonomous vehicles. For example, Tesla predominantly used radar and camera sensors for their features like Autopilot, and did not use LiDAR. However, sensor technology in the automotive industry evolves rapidly, so it's crucial to check the latest specifications for specific car models.
What Does Yellow Mean on Radar?
In radar imagery, yellow typically represents moderate rainfall or precipitation intensity. The color coding in radar images is used to indicate the severity of weather conditions, with different colors denoting various levels of intensity. Yellow often indicates a moderate level of precipitation, falling between light green (light rain) and red (heavy rain) on the intensity scale.
Are Police Laser Guns Accurate?
Police laser guns, also known as LIDAR (Light Detection and Ranging) speed guns, are generally known for their accuracy, typically measuring vehicle speeds within a margin of around +/- 1 mph or +/- 2 km/h. Some LIDAR models can maintain accuracy to within one mile per hour for speeds up to 60 miles per hour and within three percent for speeds exceeding 60 miles per hour. These devices are quite precise when used correctly.
However, it's important to note that LIDAR speed guns are not infallible. Factors such as excessive cloud cover, rain, wind, or fog can negatively impact their accuracy, making it more challenging for them to obtain accurate readings.
How Much Does a Dopper Radar Cost?
Doppler radars can cost anywhere from $14,000 to over $1 million. The price of a Doppler radar depends on the type of radar and its features. For example, a basic entry-level radar system can cost around $14,000, while a high-end dual-polarity system can cost over $1 million.